the Creative Commons Attribution 4.0 License.
the Creative Commons Attribution 4.0 License.
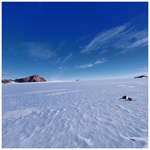
Brief communication: Identification of 140 000-year-old blue ice in the Grove Mountains, East Antarctica, by krypton-81 dating
Zhengyi Hu
Wei Jiang
Yuzhen Yan
Yan Huang
Xueyuan Tang
Lin Li
Florian Ritterbusch
Guo-Min Yang
Zheng-Tian Lu
The presence of exceptionally old ice and relative ease of access make Antarctic blue-ice areas (BIAs) attractive paleoclimate archives. However, only a handful of BIAs, mostly situated in West Antarctica and along the Transantarctic Mountains, have been investigated for this purpose. Here, we present the age of surface ice from the Grove Mountains BIA in Princess Elizabeth Land, East Antarctica, determined by measuring 81Kr in the trapped air. Two samples yield an average age of kyr. Together with the reported terrestrial age of a chondrite, we conclude that the Grove Mountains BIA holds considerable potential for paleoclimate studies.
- Article
(2026 KB) - Full-text XML
-
Supplement
(435 KB) - BibTeX
- EndNote
Antarctic ice cores provide a wealth of information about the Earth's past climate and atmospheric composition, especially greenhouse gases (e.g., Petit et al., 1999). International efforts are underway to locate and retrieve an ice core dating back 1.5 Myr that is in stratigraphic order (Fischer et al., 2013; Passalacqua et al., 2018), e.g., the ongoing Beyond EPICA Oldest Ice project at Little Dome C (https://www.beyondepica.eu/en/about/, last access: 27 February 2024). However, such endeavors are expensive and time-consuming. Shallow drilling in blue-ice areas (BIAs) in Antarctica has therefore emerged as a complementary approach (Yan et al., 2019). BIAs are regions where ablation exceeds accumulation. The negative mass balance at the surface is maintained by the supplies of crystalline glacial ice from below. In this case, ancient ice that was once buried deep in the ice sheet is brought to the surface and can be readily accessed. The presence of meteorites that have terrestrial ages of up to 2 Myr in the BIAs hints at the existence of 300 kyr old ice (Scherer et al., 1997).
In Antarctica, a number of BIAs have been investigated to understand local meteorology, glaciology, and meteorites (e.g., Spaulding et al., 2013; Liu et al., 2010; Scherer et al., 1997). Thus far, debris-free ice samples have been recovered from only five blue-ice areas for the purpose of paleoclimate studies (Table 1 and Fig. 1): Mount Moulton and Patriot Hills in West Antarctica and Allan Hills, Taylor Glacier, and Larsen Glacier in East Antarctica. All three East Antarctic BIAs are situated in Victoria Land despite the considerable presence of BIAs in other parts of East Antarctica, such as near the Grove Mountains. In this study, we focus on the Grove Mountains, which consist of a series of nunataks southwest of Princess Elizabeth Land, East Antarctica. They are approximately 400 km from the Antarctic coast and on a different side of the continent, far away from the cluster of previous sites (Fig. 1). We report the age of surface ice in the Grove Mountains determined by 81Kr dating and evaluate the potential of the Grove Mountains BIA as paleoclimate archives.
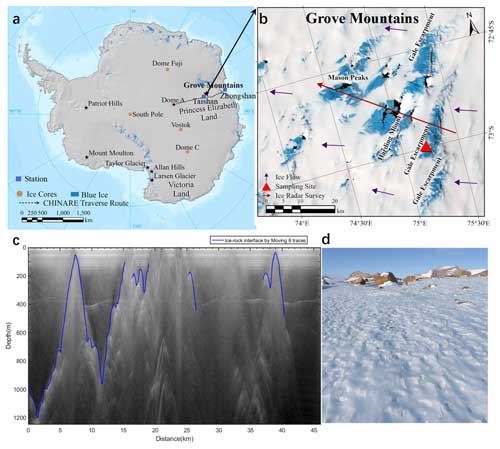
Figure 1Antarctic blue-ice areas (BIAs) and the Grove Mountains. (a) Sites of BIAs explored as paleoclimate archives listed in Table 1 (black stars), sites of deep ice coring, and the traverse route of this work from the coastal Zhongshan station to Dome A (base map from Esri). (b) Satellite imagery of the Grove Mountains BIA with local ice flow lines (purple arrows) and the transect of an airborne ice radar survey (red arrow) (base image is Landsat Image Mosaic of Antarctica). The sampling site for this study is marked with a red triangle. (c) Radar profile of the transect. (d) Surface morphology of the blue ice at the sampling site, the red triangle in panel (b) (credit: Zhengyi Hu).
2.1 Site and sample description
The Grove Mountains BIA is located in the southwest of Princess Elizabeth Land, East Antarctica (Fig. 1). Ice flows from the southeast to the northwest and eventually drains into Lambert Glacier. Ice flow is blocked by a series of nunataks in this region. The combined effect of glacial flows and topographic barriers leads to the formation of blue ice as well as a large number of crevasses that prevent access for ice drilling. The highest nunatak, Mason Peak, has an elevation of 2365 m and a topographic prominence of ∼ 700 m. The average ice elevation of the Grove Mountains is ∼ 2000 m, and past radar surveys have revealed steep bedrock topography and thick (>1000 m) ice layers (Fig. 1). The annual average temperature in the Grove Mountains is ∼ −30 °C. Similarly to other Antarctic BIAs, Grove Mountains BIA is a meteorite concentration site. To date, more than 10 000 meteorites have been recovered by the Chinese National Antarctic Research Expedition (CHINARE) from this region. In January 2016, surface blue ice (up to ∼ 50 cm in depth) was collected manually using stainless-steel spades near the mid-Gale Escarpment where the meteorites are concentrated. The surface morphology of the blue ice at the sampling site is shown in Fig. 1d. The sampling size is approximately 40 × 40 × 40 cm. The ice samples are irregular blocks with lengths ranging from about 10 to 30 cm. Based on visual inspection, there are no clear cracks and melted layers in the ice at the sampling site. In total, about 40 kg of ice was collected (72.99° S, 75.22° E). A ∼ 5 cm thick layer of surface ice was removed before sampling. The collected ice was kept in clean polyethylene bags and then preserved in insulated cabinets and transported under freezing conditions (−20 °C).
2.2 Analytical methods
The blue ice collected from the Grove Mountains was processed in two batches. The first batch (batch A) contains large ice blocks (size ∼ 20 cm or bigger) with a total weight of 26 kg. The second batch (batch B) contains small ice pieces (size 10–20 cm) and weighs about 9 kg. The reason to separate them into two batches is to see if there are more modern air contaminations in the batch with small ice samples. Before melting, the outer 3–5 mm layer of the ice was removed to reduce potential contamination from modern air. The ice was then melted in an evacuated container. The released air was transferred into 1 L stainless-steel bottles with a compressor. The extraction system typically achieves recoveries >95 % and contamination <1 ‰. The gas contents obtained for sample batch A and batch B were 95 and 86 mL STP kg−1 (STP denotes standard temperature and pressure), respectively. The difference is probably due to the larger specific surface area of the smaller sample (batch B) compared to the large one (batch A) and hence greater gas losses from smaller ice samples. Nonetheless, these gas contents are comparable to the blue ice retrieved from other BIAs in Antarctica (e.g., Buizert et al., 2014). Krypton (Kr) was separated from the extracted air with a purification system based on titanium gettering and gas chromatography (Jiang et al., 2020), yielding Kr purities and recoveries higher than 90 %. Totals of 2.2 and 0.6 µL STP Kr were obtained for the larger and smaller sample, respectively.
The Kr sample was measured with the atom trap trace analysis (ATTA) method (Jiang et al., 2020); 81Kr and 85Kr atoms are selectively captured by laser beams in a magneto-optical trap and are counted by detecting their fluorescence. Meanwhile, 83Kr (a stable isotope) is chosen as the reference and its capture rate is also measured. Each analysis took about 5 h. The measurement is cycled between the atom-counting mode for 81Kr and 85Kr and the capture-rate mode for 83Kr in order to cancel the slow drifts in the capture efficiencies of the instrument. The anthropogenic 85Kr isotope is analyzed since it has a half-life of 10.7 years, making it a good indicator of cross-sample contamination from the modern reference sample. As the amount of Kr from the ice samples is generally limited, it is important to keep the cross-sample contamination under control. This effect comes from the discharge source in the ATTA instrument. On the one hand, it slowly consumes the Kr sample in the system and makes the effective sample size smaller. On the other hand, Kr from the previous measurement is slowly released from vacuum parts to cause cross-sample contamination. In order to reduce this effect, the vacuum system of the ATTA instrument was washed continuously with a Xe discharge for 1 week. After the washing, the Kr outgassing rate was about 10−3 µL STP h−1. For a 5 h measurement the cross-sample contamination is 1 %– 3 %. The residual cross-sample contamination effect was corrected based on the 85Kr measurement.
The measured relative 81Kr abundance was used to calculate the age of the sample based on the radioactive decay law and the atmospheric input function of 81Kr. The uncertainty in the 81Kr age mainly came from the statistical errors for atom counting. The uncertainty caused by the cross-sample contamination correction was included through error propagation. Since the atom counts for both 81Kr and 85Kr were low (10–100), we adopted the Feldman–Cousins method, which provided a unified approach to treating measurements with small signals (Feldman and Cousins, 1998). Besides the statistical error, there were additional systematic errors due to the uncertainty in the half-life of 81Kr (229 ± 11 cal kyr) and the uncertainty in the atmospheric 81Kr input functions (Zappala et al., 2020). Note that the measurements were performed in 2017. The ATTA instrument has been improved significantly since then. The sample requirement is now less than 2.0 kg, and the dating precision is also better (Crotti et al., 2021).
Two pieces of blue ice from batch A were randomly selected for chemical measurements. In a Class-1000 clean room, the surface layer of ∼ 1 cm was washed with ultrapure Milli-Q water (18.2 MΩ) to remove any surface contaminants. Then the ice was melted under a super-clean hood (Class 100) at 20 °C for chemical measurements. The major chemical ions, Na+, K+, Mg2+, Cl−, NO, and SO, were determined with an ICS-3000 ion chromatography system (Dionex, USA). More details on ion analysis are provided in Shi et al. (2012). The δ18O and δD of ice were measured with a wavelength-scanned cavity ring-down spectroscopy (WS-CRDS) instrument, Picarro L2130-i (Picarro Inc., USA), with analysis precision of 0.05 ‰ and 0.5 ‰, respectively. Details on the water isotope analysis were described in a previous study (Ma et al., 2020).
2.3 Ice radar survey
During CHINARE-36 (2019–2020), radar data were collected in the Grove Mountains using an ice radar system mounted on the Snow Eagle 601 fixed-wing aircraft. The length of the radar line is about 45 km (Fig. 1b). During data acquisition, the system emits electromagnetic waves with a central frequency of 60 MHz, with a peak emission power of 8 kW and a pulse frequency of 6250 Hz. The radar antenna system consists of two flat dipole antennas that are mounted below the aircraft wing and used for both transmission and reception.
The results of radiometric Kr dating of two batches of ice from the Grove Mountains BIAs are shown in Table 2. The isotopic abundances of 81Kr in the trapped air of the two batches are statistically indistinguishable from each other. Furthermore, despite the different ice sizes and weights, both batches show a non-zero but similar level of 85Kr activities, indicating modern air contaminations possibly due to cracks near the blue-ice surface. Similar intrusion of the modern atmosphere to the blue ice has previously been observed in other BIAs as well (Spaulding et al., 2013). After correcting for the modern air contamination (assuming an atmospheric 85Kr activity of 70 ± 5 decay per minute per cubic centimeter of krypton at STP (dpm cm−3) at the sampling time (25 January 2016); Kersting et al., 2020), the averaged relative is 65 ± 6 (percent modern krypton, pMKr), which corresponds to an age of kyr. This age is comparable to the previously reported age of the surface ice in Mount Moulton, Patriot Hills, and Allan Hills BIAs (Korotkikh et al., 2011; Spaulding et al., 2013; Turney et al., 2020). Moreover, a Renazzo-type chondrite (no. GRV 021710) discovered near the sampling site has a terrestrial age of 260 kyr (Lu, 2008). These results hint at the presence of even older blue ice in the vicinity of the Grove Mountains.
The mean concentrations of Na+, K+, Mg2+, Cl−, NO, and SO in the samples are 34.9, 9.2, 11.1, 84.0, 44.4, and 94.5 ng g−1, respectively, which are similar to the values of surface snow samples collected along the Chinese inland Antarctica traverse route, about ∼ 60 km from the study site (Shi et al., 2021). The mean δ18O and δ2H of the blue ice are −40.3 ‰ and −321.2 ‰, respectively, also similar to those of the nearby surface snow (Ma et al., 2020). These stable water isotope values are much higher than those of the snow and ice on the East Antarctic plateau today (Xiao et al., 2008, and references therein) (Table S1) and during the Last Interglacial period (e.g., Petit et al., 1999). Assuming no isotopic modifications after snow deposition and during ice flow, the original deposition site of the surface ice at the Grove Mountains BIA today was likely local, but we acknowledge that the exact location remains unknown. Any future attempt to interpret those isotope data in the context of paleoclimate would require a more thorough investigation of the provenance of the blue ice.
The mean age of two surface ice samples from the Grove Mountains BIA, dated radiometrically by 81Kr, is kyr; that is, the ice dates back to the Last Interglacial, holding important implications for paleoclimate studies. Furthermore, a meteorite (GRV 021710) that has a terrestrial age of 260 kyr suggests that the Grove Mountains BIA could harbor even older ice. In general, the major chemical ions and stable water isotopes of the ice resemble those of the nearby surface snow and differ from those from Antarctic plateau sites, suggesting that the blue ice originated nearby. There are no previously published deep-ice-core records from Princess Elizabeth Land that date back to the Last Interglacial. Consequently, the Grove Mountains BIA holds the potential to provide large-volume ice samples to study the climate variations during the Last Interglacial in the Indian Ocean sector of Antarctica. To obtain old ice, the potential drilling sites in the BIAs are usually located upstream of the ice flow, where the ice stream was blocked by the nunataks, similarly to at the drilling sites in Allan Hills (Yan et al., 2019). Accordingly, the potential old-ice drilling sites in the Grove Mountains BIA are expected to be around the mid-Gale Escarpment, following the ice flow direction in this region (Fig. 1). It is noted that there are a large number of ice crevasses that have formed on the side of the mid-Gale Escarpment facing the ice flow, making it currently inaccessible from the ground. The radar profile provides direct observations of deep englacial stratigraphy in this BIA (Fig. 1c). However, only some disturbed layers can be imaged, at depths of 200–400 m beneath the ice surface. The radar image showed that the internal layers are not well identified at a depth of >500 m, which could be the result of complex ice flow patterns around the nunataks. Nonetheless, it can be seen that the subglacial topographic mountains may cause the ice to flow toward the surface, especially near the nunataks, where the ice depth is relatively shallow, at a few hundred meters. These areas are expected to be potential shallow ice core drilling sites, i.e., providing easier access to the oldest ice. In addition to retrieving ice cores, a synergistic effect of drilling operations in the Grove Mountains is the potential recovery of bedrock samples. Some previous studies have suggested the East Antarctic Ice Sheet retreated beyond the Grove Mountains during past warm intervals (Liu et al., 2010). Bedrock samples from the Grove Mountains can help evaluate this hypothesis and improve our understanding of ice sheet behaviors in a fast-warming world.
In addition, the bedrock at the Grove Mountains BIA could reveal important information about the stability of the East Antarctic Ice Sheet in past interglacials. Given these considerations, we conclude that the Grove Mountains comprise a region with high scientific value. Future drilling operations in the Grove Mountains BIA could also benefit from its close proximity to a nearby Antarctic research base (the Chinese Taishan Station; Fig. 1a). More systematic glaciological surveys in this region are called for, with the ultimate goal of retrieving ice cores and bedrock samples to study paleoclimate and past ice sheet behaviors.
Data presented in this work are included in the main text (Table 2).
The supplement related to this article is available online at: https://doi.org/10.5194/tc-18-1647-2024-supplement.
GS and ZH conceived the study. YY, WJ, and GS designed and wrote the manuscript with the support of all co-authors. ZH and GS analyzed ions and stable water isotopes. WJ, FR, GMY, and ZTL analyzed the Kr data. YH, XT, LL, and GS prepared the figure.
The contact author has declared that none of the authors has any competing interests
Publisher’s note: Copernicus Publications remains neutral with regard to jurisdictional claims made in the text, published maps, institutional affiliations, or any other geographical representation in this paper. While Copernicus Publications makes every effort to include appropriate place names, the final responsibility lies with the authors.
This article is part of the special issue “Oldest Ice: finding and interpreting climate proxies in ice older than 700 000 years (TC/CP/ESSD inter-journal SI)”. It is not associated with a conference.
This work was supported by the National Science Foundation of China (grant nos. 42276243, 41922046, 42071306, T2325024, and 41727901). The authors are grateful to CHINARE members for their support and assistance in sample collection.
This research has been supported by the National Natural Science Foundation of China (grant nos. 42276243, 41922046, 42071306, T2325024, and 41727901).
This paper was edited by Chris R. Stokes and reviewed by two anonymous referees.
Buizert, C., Baggenstos, D., Jiang, W., Purtschert, R., Petrenko, V. V., Lu, Z.-T., Müller, P., Kuhl, T., Lee, J., Severinghaus, J. P., and Brook, E. J.: Radiometric 81Kr dating identifies 120,000-year-old ice at Taylor Glacier, Antarctica, P. Natl. Acad. Sci. USA, 111, 6876–6881, https://doi.org/10.1073/pnas.1320329111, 2014.
Crotti, I., Landais, A., Stenni, B., Bazin, L., Parrenin, F., Frezzotti, M., Ritterbusch, F., Lu, Z.-T., Jiang, W., Yang, G.-M., Fourré, E., Orsi, A., Jacob, R., Minster, B., Prié, F., Dreossi, G., and Barbante, C.: An extension of the TALDICE ice core age scale reaching back to MIS 10.1, Quaternary Sci. Rev., 266, 107078, https://doi.org/10.1016/j.quascirev.2021.107078, 2021.
Feldman, G. J. and Cousins, R. D.: Unified approach to the classical statistical analysis of small signals, Phys. Rev. D, 57, 3873–3889, https://doi.org/10.1103/PhysRevD.57.3873, 1998.
Fischer, H., Severinghaus, J., Brook, E., Wolff, E., Albert, M., Alemany, O., Arthern, R., Bentley, C., Blankenship, D., Chappellaz, J., Creyts, T., Dahl-Jensen, D., Dinn, M., Frezzotti, M., Fujita, S., Gallee, H., Hindmarsh, R., Hudspeth, D., Jugie, G., Kawamura, K., Lipenkov, V., Miller, H., Mulvaney, R., Parrenin, F., Pattyn, F., Ritz, C., Schwander, J., Steinhage, D., van Ommen, T., and Wilhelms, F.: Where to find 1.5 million yr old ice for the IPICS “Oldest-Ice” ice core, Clim. Past, 9, 2489–2505, https://doi.org/10.5194/cp-9-2489-2013, 2013.
Jiang, W., Hu, S.-M., Lu, Z.-T., Ritterbusch, F., and Yang, G.-M.: Latest development of radiokrypton dating – A tool to find and study paleogroundwater, Quatern. Int., 547, 166–171, https://doi.org/10.1016/j.quaint.2019.04.025, 2020.
Kersting, A., Schlosser, C., Bollhöfer, A., and Suckow, A.: Evaluating 5 decades of atmospheric 85Kr measurements in the southern hemisphere to derive an input function for dating water and ice with implications for interhemispheric circulation and the global 85Kr emission inventory, J. Environ. Radioactiv., 225, 106451, https://doi.org/10.1016/j.jenvrad.2020.106451, 2020.
Korotkikh, E. V., Mayewski, P. A., Handley, M. J., Sneed, S. B., Introne, D. S., Kurbatov, A. V., Dunbar, N. W., and McIntosh, W. C.: The last interglacial as represented in the glaciochemical record from Mount Moulton Blue Ice Area, West Antarctica, Quaternary Sci. Rev., 30, 1940–1947, https://doi.org/10.1016/j.quascirev.2011.04.020, 2011.
Lee, G., Ahn, J., Ju, H., Ritterbusch, F., Oyabu, I., Buizert, C., Kim, S., Moon, J., Ghosh, S., Kawamura, K., Lu, Z.-T., Hong, S., Han, C. H., Hur, S. D., Jiang, W., and Yang, G.-M.: Chronostratigraphy of the Larsen blue-ice area in northern Victoria Land, East Antarctica, and its implications for paleoclimate, The Cryosphere, 16, 2301–2324, https://doi.org/10.5194/tc-16-2301-2022, 2022.
Liu, X., Huang, F., Kong, P., Fang, A., Li, X., and Ju, Y.: History of ice sheet elevation in East Antarctica: Paleoclimatic implications, Earth Planet. Sci. Lett., 290, 281–288, https://doi.org/10.1016/j.epsl.2009.12.008, 2010.
Lu, R.: Classification and study of cosmogenic 10Be and 26Al of Antarctic meteorites collected from Grove Mountains region, PhD, Institute of Geochemistry, Chinese Academy of Sciences, Guiyang, 91 pp., https://d.wanfangdata.com.cn/thesis/Y1620889 (last access: 1 February 2024), 2008 (in Chinese with English abstract).
Ma, T., Li, L., Li, Y., An, C., Yu, J., Ma, H., Jiang, S., and Shi, G.: Stable isotopic composition in snowpack along the traverse from a coastal location to Dome A (East Antarctica): Results from observations and numerical modeling, Polar Sci., 24, 100510, https://doi.org/10.1016/j.polar.2020.100510, 2020.
Passalacqua, O., Cavitte, M., Gagliardini, O., Gillet-Chaulet, F., Parrenin, F., Ritz, C., and Young, D.: Brief communication: Candidate sites of 1.5 Myr old ice 37 km southwest of the Dome C summit, East Antarctica, The Cryosphere, 12, 2167–2174, https://doi.org/10.5194/tc-12-2167-2018, 2018.
Petit, J. R., Jouzel, J., Raynaud, D., Barkov, N. I., Barnola, J. M., Basile, I., Bender, M., Chappellaz, J., Davis, M., Delaygue, G., Delmotte, M., Kotlyakov, V. M., Legrand, M., Lipenkov, V. Y., Lorius, C., PÉpin, L., Ritz, C., Saltzman, E., and Stievenard, M.: Climate and atmospheric history of the past 420,000 years from the Vostok ice core, Antarctica, Nature, 399, 429–436, https://doi.org/10.1038/20859, 1999.
Scherer, P., Schultz, L., Neupert, U., Knauer, M., Neumann, S., Leya, I., Michel, R., Mokos, J., Lipschutz, M. E., Metzler, K., Suter, M., and Kubik, P. W.: Allan Hills 88019: An Antarctic H-chondrite with a very long terrestrial age, Meteorit. Planet. Sci., 32, 769–773, https://doi.org/10.1111/j.1945-5100.1997.tb01567.x, 1997.
Shi, G., Li, Y., Jiang, S., An, C., Ma, H., Sun, B., and Wang, Y.: Large-scale spatial variability of major ions in the atmospheric wet deposition along the China Antarctica transect (31° N–69° S), Tellus B, 64, 17134, https://doi.org/10.3402/tellusb.v64i0.17134, 2012.
Shi, G., Ma, H., Hu, Z., Chen, Z., An, C., Jiang, S., Li, Y., Ma, T., Yu, J., Wang, D., Lu, S., Sun, B., and Hastings, M. G.: Brief communication: Spatial and temporal variations in surface snow chemistry along a traverse from coastal East Antarctica to the ice sheet summit (Dome A), The Cryosphere, 15, 1087–1095, https://doi.org/10.5194/tc-15-1087-2021, 2021.
Spaulding, N. E., Higgins, J. A., Kurbatov, A. V., Bender, M. L., Arcone, S. A., Campbell, S., Dunbar, N. W., Chimiak, L. M., Introne, D. S., and Mayewski, P. A.: Climate archives from 90 to 250 ka in horizontal and vertical ice cores from the Allan Hills Blue Ice Area, Antarctica, Quaternary Res., 80, 562–574, https://doi.org/10.1016/j.yqres.2013.07.004, 2013.
Turney, C. S., Fogwill, C. J., Golledge, N. R., McKay, N. P., van Sebille, E., Jones, R. T., Etheridge, D., Rubino, M., Thornton, D. P., and Davies, S. M.: Early Last Interglacial ocean warming drove substantial ice mass loss from Antarctica, P. Natl. Acad. Sci. USA, 117, 3996–4006, https://doi.org/10.1073/pnas.1902469117, 2020.
Xiao, C., Li, Y., Hou, S., Allison, I., Bian, L., and Ren, J.: Preliminary evidence indicating Dome A (Antarctica) satisfying preconditions for drilling the oldest ice core, Chin. Sci. Bull., 53, 102–106, https://doi.org/10.1007/s11434-007-0520-6, 2008.
Yan, Y., Bender, M. L., Brook, E. J., Clifford, H. M., Kemeny, P. C., Kurbatov, A. V., Mackay, S., Mayewski, P. A., Ng, J., Severinghaus, J. P., and Higgins, J. A.: Two-million-year-old snapshots of atmospheric gases from Antarctic ice, Nature, 574, 663–666, https://doi.org/10.1038/s41586-019-1692-3, 2019.
Zappala, J. C., Baggenstos, D., Gerber, C., Jiang, W., Kennedy, B. M., Lu, Z. T., Masarik, J., Mueller, P., Purtschert, R., and Visser, A.: Atmospheric 81Kr as an Integrator of Cosmic-Ray Flux on the Hundred-Thousand-Year Time Scale, Geophys. Res. Lett., 47, e2019GL086381, https://doi.org/10.1029/2019GL086381, 2020.