the Creative Commons Attribution 4.0 License.
the Creative Commons Attribution 4.0 License.
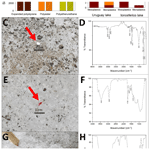
A pilot study about microplastics and mesoplastics in an Antarctic glacier
Miguel González-Pleiter
Gissell Lacerot
Carlos Edo
Juan Pablo Lozoya
Francisco Leganés
Francisca Fernández-Piñas
Roberto Rosal
Franco Teixeira-de-Mello
Plastics have been found in several compartments in Antarctica. However, there is currently no evidence of their presence on Antarctic glaciers. Our pilot study investigated plastic occurrence on two ice surfaces (one area around Uruguay Lake and another one around Ionosferico Lake) that constitute part of the ablation zone of Collins Glacier (King George Island, Antarctica). Our results showed that expanded polystyrene (EPS) was ubiquitous, ranging from 0.17 to 0.33 items m−2, whereas polyester was found only on the ice surface around Uruguay Lake (0.25 items m−2). Furthermore, we evaluated the daily changes in the presence of plastics in these areas in the absence of rainfall to clarify the role of the wind in their transport. We registered an atmospheric dry deposition rate between 0.08 items m−2 d−1 on the ice surface around Uruguay Lake and 0.17 items m−2 d−1 on the ice surface around Ionosferico Lake. Our pilot study is the first report of plastic pollution presence on an Antarctic glacier, possibly originated from local current and past activities and likely deposited by wind transport.
- Article
(3333 KB) - Full-text XML
-
Supplement
(507 KB) - BibTeX
- EndNote
The cryosphere is the frozen water part of the Earth system that consists of areas in which the temperatures are below 0 ∘C for at least part of the year (NOAA, 2019). Most of the cryosphere in terms of volume of ice is in Antarctica. It has been estimated that the Antarctic cryosphere holds around 90 % of Earth's ice mass (Dirscherl et al., 2020), with an increasing rate of ice loss during the last decades (Rignot et al., 2019). Furthermore, the Antarctic cryosphere represents the majority of the world's fresh water, representing the largest freshwater ecosystem on the planet (Shepherd et al., 2018).
Plastics, especially microplastics (plastic items <5 mm long; MPs), have been detected in several specific locations of the cryosphere, including mountain glaciers (Ambrosini et al., 2019; Cabrera et al., 2020; Matericì et al., 2020), polar and urban snow (Bergmann et al., 2019; Österlund et al., 2019), and sea ice (Geilfus et al., 2019; Kelly et al., 2020; La Daana et al., 2020; Obbard et al., 2014; Peeken et al., 2018; von Friesen et al., 2020). The occurrence of MPs in snow ranged from 0 to 1.5×105 MPs L−1 of melted snow (Bergmann et al., 2019), although it should be noted that a part of this study was conducted near urban areas. Regarding sea ice, concentrations of up to 1.2×104 MPs L−1 have been reported, although there are large differences between studies even from the same region (Peeken et al., 2018; von Friesen et al., 2020). The use of different units in reporting MP concentrations in mountain glaciers such as the number of items per mass of ice weight (78.3 ± 30.2 MPs kg−1 of sparse and fine supraglacial debris; Ambrosini et al., 2019) and mass of MPs per volume (0 to 23.6 ± 3.0 ng of MPs mL−1; Matericì et al., 2020) makes comparisons between studies difficult (e.g., 101.2 items L−1; Cabrera et al., 2020). Regarding the shape of the MPs found in the cryosphere, fibers seem to be dominant in mountain glaciers (65 %) and sea ice (79 %), followed by fragments (Ambrosini et al., 2019; La Daana et al., 2020). Concerning the size of MPs, a broad size distribution in sea ice has been reported, with 67 % of MPs in the 500–5000 µm range (La Daana et al., 2020). Other studies found lower sizes, however, with significant amounts (up to 90 %) of MPs smaller than 100 µm in snow and sea ice (Ambrosini et al., 2019; Bergmann et al., 2017, 2019; Kelly et al., 2020; Peeken et al., 2018). The differences between these studies may be due to the different analytical methods used, particularly methodologies such as micro Fourier transform infrared spectroscopy (µFTIR; which can identify smaller-sized MPs). In general, the presence of plastics larger than 5 mm has not been reported in the cryosphere, probably because they occur at lower concentrations and evade detection. µFTIR revealed that polyethylene terephthalate (PET), polyamide (PA), polyester (PE), varnish (acrylates or polyurethane), several synthetic rubbers, polypropylene (PP) and polyurethane (PU) are the most common types of MPs in the cryosphere (Ambrosini et al., 2019; Bergmann et al., 2017, 2019; La Daana et al., 2020; Matericì et al., 2020; Obbard et al., 2014; Peeken et al., 2018). The sources of MPs detected in the cryosphere, however, remain poorly understood. It has been suggested that they could be transported by the wind before being deposited by both wet and dry deposition in remote areas such as polar regions (Halsband and Herzke, 2019). In fact, it has been reported that air masses can transport MPs through the atmosphere over distances of at least 100 km and that they can be released from the marine environment into the atmosphere by sea spray (Allen et al., 2020, 2019; González-Pleiter et al., 2020a).
So far, plastics have been found in specific parts of the cryosphere (mountain glacier, snow and sea ice) and Antarctica (seawater, fresh water, sediments and organisms). We hypothesize that plastics have also reached freshwater glaciers in Antarctica and that wind transport plays a crucial role in this process. To test this hypothesis, we carried out a pilot study to investigate the presence of plastics on two ice surfaces (an area around Uruguay Lake and another one around Ionosferico Lake) that constitute part of the ablation zone of Collins Glacier in Maxwell Bay on King George Island (Antarctica). Furthermore, the daily changes in the presence of plastics in these ice surfaces were evaluated in the absence of precipitation to clarify the role of wind in their transport.
2.1 Study area
Collins Glacier is located in the northeast of Fildes Peninsula (King George Island, Antarctica; Fig. 1a) and has a total surface area of 15 km2 (Simoes et al., 2015). Our study was carried out on the ice surface of the glacier ablation areas around two lakes (Uruguay or Profound and Ionosferico) in Maxwell Bay (Fig. 1b). Uruguay Lake (62∘11′6.54′′ S, 58∘54′42.23′′ E) is located in the proximity of the Artigas Antarctic Scientific Base, and its access road (∼ 300 m) is subjected to human transit (Fig. 1b). The distance from the shoreline to Uruguay Lake is ∼ 366 m. The lake is used for drinking and domestic water supply. The glacier surface studied in this lake covered 1680 m2. Ionosferico Lake (62∘11′59.41′′ S, 58∘57′44.17′′ E) is located ∼ 600 m from Artigas Base and has minimal human activity. The distance from the shoreline to Ionosferico Lake is ∼ 694 m. The glacier surface studied covered 537 m2 (Fig. 1b). It should be noted that there were no visible footpaths through or nearby the glacier surfaces of both lakes during the duration of our study (except our own footprints).
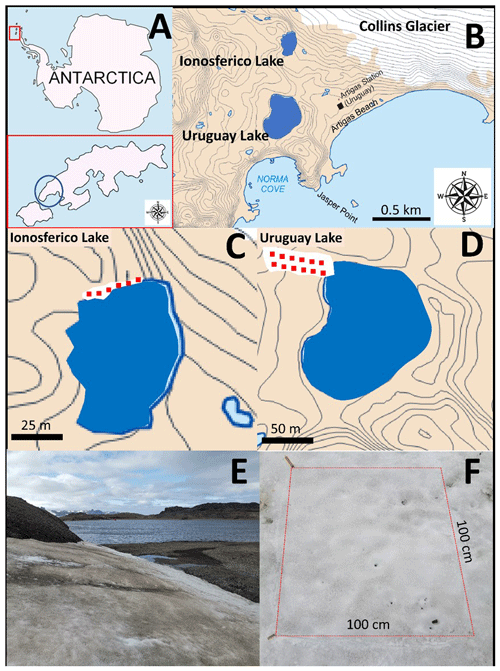
Figure 1(a) General view of Antarctica and location of King George Island. The blue ellipse indicates the Fildes Peninsula. Collins Glacier is located in the northeast of Fildes Peninsula. (b) A detailed view of Ionosferico Lake, Uruguay Lake, Artigas Research Station and Collins Glacier in the Fildes Peninsula. Panels (c) and (d) show the ablation zone of Collins Glacier around Ionosferico Lake and Uruguay Lake, respectively. Red squares indicate sampling squares. (e) Photograph of the glacier surface around Uruguay Lake that constitutes part of the ablation zone of Collins Glacier taken on 18 February 2020. (f) A representative square on the glacier surface used in this study.
2.2 Experimental assessment of plastic concentration
To evaluate the concentration of plastics, 12 squares were marked on the ice surface around Uruguay Lake (Fig. 1c) and 6 squares on the ice surface around Ionosferico Lake (Fig. 1d), which constitute part of the ablation zone of Collins Glacier, on 18 February 2020. The first square of 1 m2 on the ice surface on each lake was randomly marked. After that, the rest of the squares of 1 m2 were distributed every 10 m, covering the entire ice surface around each lake (Fig. 1d). All items visually resembling plastic (suspected plastic) inside the squares were registered (Fig. 1f). It should be noted that our sampling strategy excluded the plastics undetectable by the naked eye (i.e., small plastics such as fibers). Thus, we probably underestimated the concentration of small plastics on the ice surface.
2.3 Experimental assessment of atmospheric dry deposition of plastics
After the initial sampling, we selected six squares on the ice on each lake for subsequent daily monitoring. Additional sampling was performed every 12 h for 2 d (18 and 20 February 2020) after the initial sampling. No precipitation occurred during the duration of the experiment.
2.4 Characterization and identification of plastics
Every item visually resembling plastic detected in the squares was collected with stainless-steel tweezers, placed into glass bottles and stored at 4 ∘C until analysis. All collected items were photographed and measured, and their composition was identified by attenuated total reflectance Fourier transform infrared spectroscopy (ATR-FTIR) using an Agilent Cary 630 FTIR spectrometer or by µFTIR on a Perkin-Elmer Spotlight 200 Spectrum Two apparatus equipped with a mercury cadmium telluride (MCT) detector (depending on the size of the item). The spectra were taken using the following parameters in micro-transmission mode: spot 50 µm, 32 scans and spectral range 550–4000 cm−1 with 8 cm−1 resolution. The spectra were processed using OMNIC software (Thermo Fisher). Items with matching values >60 % were considered plastic materials. The results of concentration and atmospheric dry deposition of plastics reported in this study include only items positively identified as plastics according to the FTIR analysis and were expressed as number of items per surface unit and items per surface unit and day, respectively.
2.5 Prevention of procedural contamination
To avoid sample contamination, all materials used were previously cleaned with Milli-Q water, wrapped in aluminum foil and heated to 300 ∘C for 4 h to remove organic matter. The use of any plastic material during sampling was avoided. Furthermore, possible contamination from our clothes was controlled throughout the sampling by checking fibers and fragments extracted from the clothes against the MPs and mesoplastics (MePs) found in the samples and by positioning ourselves downwind from the sampled area. Given their size, plastics found in this study were detected by the naked eye, and their traceability could be easily maintained during quantification and identification of the samples.
3.1 Characterization and identification of the plastics
In total, 45 items preliminarily identified as plastics were collected, of which 29 items were confirmed as plastic by FTIR or µFTIR analyses (matching >60 %). The size of plastics ranged from 2292 to 12 628 µm in length and from 501 to 11 334 µm in width (Fig. 2a). According to their size, 13 mesoplastic items (plastic items between 5–25 mm long; MeP) and 3 MP items were found on the ice around Uruguay Lake and 12 MeP items and 1 MP item on the ice around Ionosferico Lake (Fig. 2b). Mesoplastics and MPs (hereinafter referred to as plastics) of expanded polystyrene (EPS) were found on the ice around both lakes: 8 plastic items on the ice around Uruguay Lake and 13 plastic items on the ice around Ionosferico Lake (Fig. 2b, c and d). Polyester (n=7 items; Fig. 2b, e and f) and polyurethane (n=1 item; Fig. 2b, g and h) items were present only on the ice around Uruguay Lake. It should be noted that spectra of the polyester (Fig. 2f) showed a high similarity with alkyd resin, a thermoplastic polyester widely used in synthetic paints.
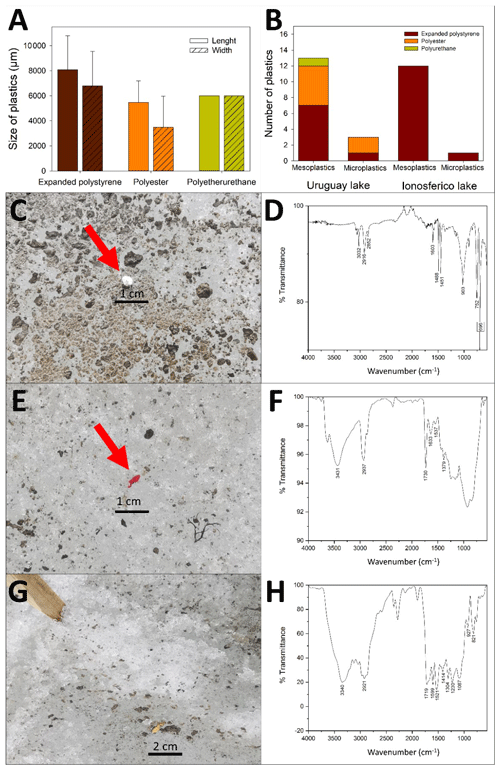
Figure 2(a) Size of the plastics collected on the glacier surface. (b) Total number of the mesoplastics and microplastics found on the glacier surface around both lakes. Representative photographs of expanded polystyrene (c), polyester (e) and polyurethane (g) found on the glacier surface. The red arrows indicate the plastics. FTIR representative spectra of expanded polystyrene (d), polyester (f) and polyurethane (h) found on the glacier surface.
3.2 Plastic concentration
EPS items were ubiquitous on the ice, with concentrations ranging from 0.17 items m−2 on the ice around Uruguay Lake to 0.33 items m−2 on the ice around Ionosferico Lake (Table S1). The concentration of polyester, which was found only on the ice around Uruguay Lake, was 0.25 items m−2 (Table S1). Polyurethane items were not observed in Ionosferico Lake (Table S1).
3.3 Atmospheric dry deposition of plastics
The dry deposition rate of EPS was 0.08 EPS and 0.17 EPS items m−2 d−1on the ice around Uruguay and Ionosferico lakes, respectively (Table S2 and Fig. 3).
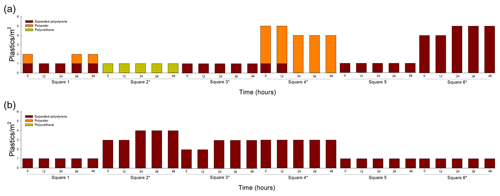
Figure 3Changes in the presence of plastics into the squares marked on ice surface around Uruguay Lake (a) and around Ionosferico Lake (b) that constitute part of the ablation zone of Collins Glacier in Maxwell Bay on King George Island (Antarctica). Plastics were monitored every 12 h for 2 d (18 and 20 February 2020) in the absence of precipitation. Asterisks indicate squares different from those used in the assessment of plastic concentration.
Polyester was only deposited on the ice around Uruguay Lake at a rate of 0.08 items m−2 d−1. Polyurethane items were not observed in Ionosferico Lake during the duration of the experiment (Table S2). The plastics deposited on the ice of Ionosferico Lake during the experiment were exclusively EPS (Table S2 and Fig. 3).
The presence of plastics has been documented in different places in Antarctica: marine surface waters (Cincinelli et al., 2017; Isobe et al., 2017; Jones-Williams et al., 2020; Lacerda et al., 2019; Suaria et al., 2020), marine sediments (Cunningham et al., 2020; Munari et al., 2017; Reed et al., 2018), zooplankton samples from ocean water (Absher et al., 2019), marine benthic invertebrates (Sfriso et al., 2020), Antarctic Collembola (Bergami et al., 2020b), penguins (Bessa et al., 2019), seabirds (Ibañez et al., 2020) and fresh water (González-Pleiter et al., 2020b). However, there was only one study showing the occurrence of plastics in the Antarctic cryosphere, which was carried out on sea ice (Kelly et al., 2020). Thus, this is the first report on the presence of MPs and MePs on the surface of Antarctic glaciers. Furthermore, our findings provide insight into the role of wind in the transport of this material.
In this sense, winds (especially high-speed ones) appear to be a key element in the transport of plastics to Antarctic glaciers. The prevailing winds in the study area (Fig. 1b) blow predominantly from the west (Fig. 4a). However, strong winds (Fig. 4b), wind gusts (Fig. 4c) and strong wind gusts (Fig. 4d) blow mainly from the east and southeast directions and could be responsible for the spreading of plastics from the different origins to the surface of the glacier ablation areas. These strong winds would explain the presence of MePs despite their size (Fig. 2a). In fact, the low density of the MePs found (mainly EPS; Fig. 2b) would have allowed their easy dispersion by wind.
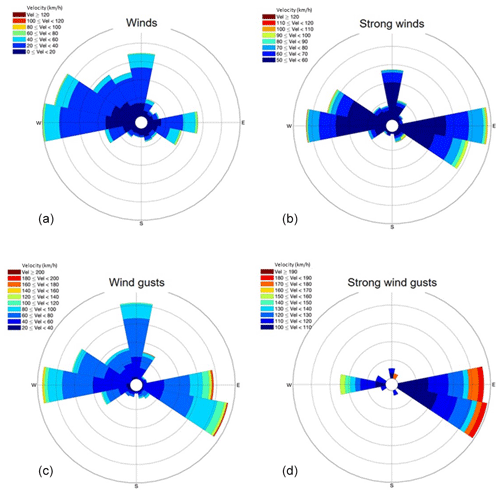
Figure 4Wind roses obtained for the area of Artigas Scientific Antarctic Base based on historical data of the Uruguayan National Institute of Meteorology (January 1998–May 2016; 24 698 records). The data are available for research through https://www.inumet.gub.uy/ (last access: 15 December 2017) with previous authorization from the institution. Based on the speed of winds considered, (a) and (b) refer to winds and strong winds, and (c) and (d) refer to wind gusts and strong wind gusts, respectively.
Our results on the wind transport and deposition of plastics support the hypothesis that the role of the wind is relevant for the short-range transport of MPs and MePs in (and among) different areas of Antarctica. The dry deposition of plastics (Table S2) was closely related to the wind regimes during the study period (Fig. S1). Based on information available on the meteorological conditions during the study dates (18–20 February 2020) in Villa Las Estrellas (Fig. S1a), which is located near Artigas Beach (Fig. S1b), the wind blew from the northeast, veering to the south with a speed between 10 and 30 km h−1 (Fig. S1a). These wind conditions suggest a possible link with the marine environment, which can act as a source of plastics (Allen et al., 2020) and potentially explain the presence of plastics on the glacier ablation areas. However, considering the low intensity of the winds recorded during those days (Fig. S1a) and the presence of MePs, it is also possible that the predominant high-speed winds transported MePs from other adjacent areas of the Fildes Peninsula to the vicinity of the lakes in the days prior to our study (Fig. 4b, c and d), and then the milder winds registered during the sampling days (Fig. S1a) deposited these MePs on the ice.
The chemical composition of the plastics found (Fig. 2d, f and h) supports the fact that the source of the plastics could be of marine and/or land-based origin. The types of plastics found (Fig. 2b) are related to human activities in the Fildes Peninsula that could generate plastic debris such as tourism, leaks in waste management at scientific bases or the presence of abandoned infrastructures. Considering the location of Collins Glacier and the main human activities on the Fildes Peninsula (e.g. airfield, scientific bases), the prevailing winds from the west could have transported small and lightweight plastics to the study area. In fact, EPS is widely used in packaging and as insulation material in old buildings in this area, and polyester is also a component of old buildings paints. In the same way, some of these plastics could be released from the marine environment to the Artigas Beach area and then be transported by the wind to the glaciers. In this sense, polyurethane MePs (which are similar to those found in this work) have already been reported in sea surface waters in the Antarctic (Jones-Williams et al., 2020), and EPS MePs have been found on Artigas Beach (Laganà et al., 2019). These findings highlight a potential threat to the fragile Antarctic ecosystem since the presence of these plastics (e.g., polystyrene particles) has been shown to affect Antarctic biota (Bergami et al., 2019, 2020a).
The role of the atmospheric dry deposition in the presence of plastics on glaciers is supported by recent studies suggesting that MPs can be transported up to hundreds of kilometers through the atmosphere before being deposited (González-Pleiter et al., 2020a). Our results showed that the atmospheric deposition of plastics on glaciers is still low, with figures between 2 and 4 orders of magnitude lower than values reported in populated areas (Brahney et al., 2020; Cai et al., 2017; Dris et al., 2016; Klein and Fischer, 2019; Roblin et al., 2020; Wright et al., 2020). Our results also show that plastic pollution, even if only in small quantities, reaches remote areas with few human settlements. The occurrence of plastic pollution in Antarctica represents the spreading of anthropogenic pollutants in the last pristine environment on the Earth, most likely linked to their presence on-site. Further research is needed then to elucidate the occurrence, sources, fate and impact of plastics in such remote places.
Taken together, our research indicates that human activities in sensitive remote areas such as Antarctica leave a footprint that includes plastic pollution. Since the early reports of litter pollution on the seafloor (Dayton and Robilliard, 1971) and, subsequently, on beaches and seabirds of Antarctica (Convey et al., 2002; Creet et al., 1994; Fijn et al., 2012; Lenihan et al., 1990; Sander et al., 2009), the handling of waste has been improved by the implementation of the Antarctic Treaty System, Annex III “Waste Disposal and Waste Management”. The treaty requires the removal of all plastic from Antarctica, with the only exception of plastics that can be incinerated without producing harmful emissions (Antarctic Treaty Secretariat, 1998). However, once plastics are broken down into smaller fractions and dispersed throughout the continent and nearby waters, management measures become very difficult to address, as evidenced by our data. Thus, a more rigorous management of plastics is essential for preserving a clean environment within the treaty area (Zhang et al., 2020).
This is the first report of the presence of both MePs and MPs on an Antarctic glacier, which were probably transported by wind from local sources such as beach areas. In total, three types of plastics (EPS, PU and polyester) were found on two glacier surfaces that constitute part of the ablation zone of Collins Glacier (King George Island, Antarctica). EPS was ubiquitous in the two glacier surfaces studied. Our study showed that the management of plastic contamination in Antarctica should be improved, focusing on the waste generated by current and past anthropogenic activities that occur in that area.
All data are shown in the paper and/or Supplement except those used to produce Fig. 4, which are available at https://www.inumet.gub.uy/ (inumet, 2017).
The supplement related to this article is available online at: https://doi.org/10.5194/tc-15-2531-2021-supplement.
MGP identified the research question, formulated the hypothesis, developed the experimental design, planned the experiments, performed the experiments in the field, performed the experiments in the laboratory, compiled the data sets, analyzed the data, discussed the results, prepared graphical material, wrote the paper (original draft) and provided financial support. GL identified the research question, formulated the hypothesis, developed the experimental design, planned the experiments, checked the field data, discussed the results and wrote the paper (final version). CE performed the experiments in the laboratory, compiled the data sets, analyzed the data, discussed the results, prepared graphical material and reviewed the final paper. JPL developed the experimental design, checked the field data, discussed the results, reviewed the final paper and provided financial support. FL discussed the results, reviewed the final paper and provided financial support. FFP checked the field data, checked the laboratory data, discussed the results, reviewed the final paper and provided financial support. RR checked the field data, checked the laboratory data, discussed the results, reviewed the final paper and provided financial support. FTdM identified the research question, formulated the hypothesis, developed the experimental design, planned the experiments, performed the experiments in the field, checked the field data, prepared graphical material, discussed the results, reviewed the final paper and provided financial support.
The authors declare that they have no conflict of interest.
This research was funded by the government of Spain (CTM2016-74927-C2-1/2-R) and the Uruguayan Antarctic Institute. Miguel González-Pleiter thanks the Carolina Foundation for the award of a postdoctoral grant (SEGIB). Carlos Edo thanks the Spanish government for the award of a predoctoral grant. The authors gratefully acknowledge the support of Fiorella Bresesti, Evelyn Krojmal and Barbara De Feo from the Centro Universitario Regional del Este, Universidad de la República, for their assistance during sampling; of Marta Elena González Mosquera from the University of Alcala for providing access to the Agilent Cary 630 FTIR spectrometer; and of Gastón Manta from Facultad de Ciencias, Universidad de la República, for providing historical wind analysis at the Artigas Antarctic Research Base. Franco Teixeira-de-Mello, Gissell Lacerot and Juan Pablo-Lozoya thank the Sistema Nacional de Investigadores (SNI) and the Programa de Desarrollo de las Ciencias Básicas (PEDEClBA).
This research has been supported by the Ministerio de Ciencia, Innovación y Universidades (grant no. CTM2016-74927-C2-1/2-R) and the Instituto Antártico Uruguayo (AntarPLAST grant).
This paper was edited by Olaf Eisen and reviewed by Melanie Bergmann, Rachel Obbard and two anonymous referees.
Absher, T. M., Ferreira, S. L., Kern, Y., Ferreira, A. L., Christo, S. W., and Ando, R. A.: Incidence and identification of microfibers in ocean waters in Admiralty Bay, Antarctica, Environ. Sci. Pollut. Res., 26, 292–298, 2019.
Allen, S., Allen, D., Phoenix, V. R., Le Roux, G., Jiménez, P. D., Simonneau, A., Binet, S., and Galop, D.: Atmospheric transport and deposition of microplastics in a remote mountain catchment, Nature Geosci., 12, 339–344, 2019.
Allen, S., Allen, D., Moss, K., Le Roux, G., Phoenix, V. R., and Sonke, J. E.: Examination of the ocean as a source for atmospheric microplastics, PLoS One, 15, e0232746, https://doi.org/10.1371/journal.pone.0232746, 2020.
Ambrosini, R., Azzoni, R. S., Pittino, F., Diolaiuti, G., Franzetti, A., and Parolini, M.: First evidence of microplastic contamination in the supraglacial debris of an alpine glacier, Environ. Pollut., 253, 297–301, 2019.
Antarctic Treaty Secretariat: Annex III to the Protocol on Environmental Protection to the Antarctic Treaty. Waste disposal and waste management, available at: http://www.ats.aq (last access: 9 February 2021), 1998.
Bergami, E., Emerenciano, A. K., González-Aravena, M., Cárdenas, C., Hernández, P., Silva, J., and Corsi, I.: Polystyrene nanoparticles affect the innate immune system of the Antarctic sea urchin Sterechinus neumayeri, Polar Biol., 42, 743–757, 2019.
Bergami, E., Manno, C., Cappello, S., Vannuccini, M., and Corsi, I.: Nanoplastics affect moulting and faecal pellet sinking in Antarctic krill (Euphausia superba) juveniles, Environ. Int., 143, 105999, https://doi.org/10.1016/j.envint.2020.105999, 2020a.
Bergami, E., Rota, E., Caruso, T., Birarda, G., Vaccari, L., and Corsi, I.: Plastics everywhere: first evidence of polystyrene fragments inside the common Antarctic collembolan Cryptopygus antarcticus, Biol. Lett., 16, 20200093, https://doi.org/10.1098/rsbl.2020.0093, 2020b.
Bergmann, M., Wirzberger, V., Krumpen, T., Lorenz, C., Primpke, S., Tekman, M. B., and Gerdts, G.: High quantities of microplastic in Arctic deep-sea sediments from the HAUSGARTEN observatory, Environ. Sci. Technol., 51, 11000–11010, 2017.
Bergmann, M., Mützel, S., Primpke, S., Tekman, M. B., Trachsel, J., and Gerdts, G.: White and wonderful? Microplastics prevail in snow from the Alps to the Arctic, Sci. Adv., 5, eaax1157, https://doi.org/10.1126/sciadv.aax1157, 2019.
Bessa, F., Ratcliffe, N., Otero, V., Sobral, P., Marques, J. C., Waluda, C. M., Trathan, P. N., and Xavier, J. C.: Microplastics in gentoo penguins from the Antarctic region, Scientific Reports, 9, 1–7, 2019.
Brahney, J., Hallerud, M., Heim, E., Hahnenberger, M., and Sukumaran, S.: Plastic rain in protected areas of the United States, Science, 368, 1257–1260, 2020.
Cabrera, M., Valencia, B. G., Lucas-Solis, O., Calero, J. L., Maisincho, L., Conicelli, B., Moulatlet, G. M., and Capparelli, M. V.: A new method for microplastic sampling and isolation in mountain glaciers: A case study of one antisana glacier, Ecuadorian Andes, Case Stud. Chem. Environ. Eng., 2, 100051, https://doi.org/10.1016/j.cscee.2020.100051, 2020.
Cai, L., Wang, J., Peng, J., Tan, Z., Zhan, Z., Tan, X., and Chen, Q.: Characteristic of microplastics in the atmospheric fallout from Dongguan city, China: preliminary research and first evidence, Environ. Sci. Pollut. Res., 24, 24928–24935, 2017.
Cincinelli, A., Scopetani, C., Chelazzi, D., Lombardini, E., Martellini, T., Katsoyiannis, A., Fossi, M. C., and Corsolini, S.: Microplastic in the surface waters of the Ross Sea (Antarctica): occurrence, distribution and characterization by FTIR, Chemosphere, 175, 391–400, 2017.
Convey, P., Barnes, D., and Morton, A.: Debris accumulation on oceanic island shores of the Scotia Arc, Antarctica, Polar Biol., 25, 612–617, 2002.
Creet, S., Van Franeker, J., Van Spanje, T., and Wolff, W.: Diet of the pintado petrel daption capense at king george island, Antarctica, 1990–91, Mar. Ornithol., 22, 221–229, 1994.
Cunningham, E. M., Ehlers, S. M., Dick, J. T., Sigwart, J. D., Linse, K., Dick, J. J., and Kiriakoulakis, K.: High abundances of microplastic pollution in deep-sea sediments: evidence from Antarctica and the Southern Ocean, Environ. Sci. Technol., 54, 13661–13671, 2020.
Dayton, P. K. and Robilliard, G. A.: Implications of pollution to the McMurdo Sound benthos, Antarctic Journal of the United States, 6, 53–56, 1971.
Dirscherl, M., Dietz, A. J., Dech, S., and Kuenzer, C.: Remote sensing of ice motion in Antarctica – A review, Remote Sens. Environ., 237, 111595, https://doi.org/10.1016/j.rse.2019.111595, 2020.
Dris, R., Gasperi, J., Saad, M., Mirande, C., and Tassin, B.: Synthetic fibers in atmospheric fallout: a source of microplastics in the environment?, Mar. Pollut. Bull., 104, 290–293, 2016.
Fijn, R. C., Van Franeker, J. A., and Trathan, P. N.: Dietary variation in chick-feeding and self-provisioning Cape Petrel Daption capense and Snow Petrel Pagodroma nivea at Signy Island, South Orkney Islands, Antarctica, Mar. Ornithol., 40, 81–87, 2012.
Geilfus, N.-X., Munson, K., Sousa, J., Germanov, Y., Bhugaloo, S., Babb, D., and Wang, F.: Distribution and impacts of microplastic incorporation within sea ice, Mar. Pollut. Bull., 145, 463–473, 2019.
González-Pleiter, M., Edo, C., Aguilera, Á., Viúdez-Moreiras, D., Pulido-Reyes, G., González-Toril, E., Osuna, S., de Diego-Castilla, G., Leganés, F., and Fernández-Piñas, F.: Occurrence and transport of microplastics sampled within and above the planetary boundary layer, Sci. Total Environ., 761, 143213, https://doi.org/10.1016/j.scitotenv.2020.143213, 2020a.
González-Pleiter, M., Edo, C., Velázquez, D., Casero-Chamorro, M. C., Leganés, F., Quesada, A., Fernández-Piñas, F., and Rosal, R.: First detection of microplastics in the freshwater of an Antarctic Specially Protected Area, Mar. Pollut. Bull., 161, 111811, https://doi.org/10.1016/j.marpolbul.2020.111811, 2020b.
Halsband, C. and Herzke, D.: Plastic litter in the European Arctic: What do we know?, Emerging Contaminants, 5, 308–318, 2019.
Ibañez, A., Morales, L., Torres, D., Borghello, P., Haidr, N., and Montalti, D.: Plastic ingestion risk is related to the anthropogenic activity and breeding stage in an Antarctic top predator seabird species, Mar. Pollut. Bull., 157, 111351, https://doi.org/10.1016/j.marpolbul.2020.111351, 2020.
inumet: https://www.inumet.gub.uy/, last access: 15 December, 2017.
Isobe, A., Uchiyama-Matsumoto, K., Uchida, K., and Tokai, T.: Microplastics in the southern ocean, Mar. Pollut. Bull., 114, 623–626, 2017.
Jones-Williams, K., Galloway, T., Cole, M., Stowasser, G., Waluda, C., and Manno, C.: Close encounters-microplastic availability to pelagic amphipods in sub-antarctic and antarctic surface waters, Environ. Int., 140, 105792, https://doi.org/10.1016/j.envint.2020.105792, 2020.
Kelly, A., Lannuzel, D., Rodemann, T., Meiners, K., and Auman, H.: Microplastic contamination in east Antarctic sea ice, Mar. Pollut. Bull., 154, 111130, https://doi.org/10.1016/j.marpolbul.2020.111130, 2020.
Klein, M. and Fischer, E. K.: Microplastic abundance in atmospheric deposition within the Metropolitan area of Hamburg, Germany, Sci. Total Environ., 685, 96–103, 2019.
Lacerda, A. L. d. F., Rodrigues, L. D. S., Van Sebille, E., Rodrigues, F. L., Ribeiro, L., Secchi, E. R., Kessler, F., and Proietti, M. C.: Plastics in sea surface waters around the Antarctic Peninsula, Scientific Reports, 9, 1–12, 2019.
La Daana, K. K., Gardfeldt, K., Krumpen, T., Thompson, R. C., and O'Connor, I.: Microplastics in sea ice and seawater beneath ice floes from the Arctic Ocean, Scientific Reports, 10, 1–11, 2020.
Laganà, P., Caruso, G., Corsi, I., Bergami, E., Venuti, V., Majolino, D., La Ferla, R., Azzaro, M., and Cappello, S.: Do plastics serve as a possible vector for the spread of antibiotic resistance? First insights from bacteria associated to a polystyrene piece from King George Island (Antarctica), Int. J. Hyg. Envir. Heal., 222, 89–100, 2019.
Lenihan, H. S., Oliver, J. S., Oakden, J. M., and Stephenson, M. D.: Intense and localized benthic marine pollution around McMurdo Station, Antarctica, Mar. Pollut. Bull., 21, 422–430, 1990.
Matericì, D. A., Kasper-Giebl, A., Kau, D., Anten, M., Greilinger, M., Ludewig, E., van Sebille, E., Ro?ckmann, T., and Holzinger, R.: Micro-and nanoplastics in Alpine Snow: a new method for chemical identification and (semi) quantification in the nanogram range, Environ. Sci. Technol., 54, 2353–2359, 2020.
Munari, C., Infantini, V., Scoponi, M., Rastelli, E., Corinaldesi, C., and Mistri, M.: Microplastics in the sediments of Terra Nova Bay (Ross Sea, Antarctica), Mar. Pollut. Bull., 122, 161–165, 2017.
NOAA: What is the cryosphere?, National Ocean Service website, 290, available at: https://oceanservice.noaa.gov/facts/cryosphere.html (last access: 15 January 2021), 2019.
Obbard, R. W., Sadri, S., Wong, Y. Q., Khitun, A. A., Baker, I., and Thompson, R. C.: Global warming releases microplastic legacy frozen in Arctic Sea ice, Earth's Future, 2, 315–320, 2014.
Österlund, H., Renberg, L., Nordqvist, K., and Viklander, M.: Micro litter in the urban environment: sampling and analysis of undisturbed snow, in: Novatech 2019 10th international conference, 1–5 July 2019, Lyon, France, 2019.
Peeken, I., Primpke, S., Beyer, B., Gütermann, J., Katlein, C., Krumpen, T., Bergmann, M., Hehemann, L., and Gerdts, G.: Arctic sea ice is an important temporal sink and means of transport for microplastic, Nat. Commun., 9, 1–12, 2018.
Reed, S., Clark, M., Thompson, R., and Hughes, K. A.: Microplastics in marine sediments near Rothera research station, Antarctica, Mar. Pollut. Bull., 133, 460–463, 2018.
Rignot, E., Mouginot, J., Scheuchl, B., Van Den Broeke, M., Van Wessem, M. J., and Morlighem, M.: Four decades of Antarctic Ice Sheet mass balance from 1979–2017, P. Natl. Acad. Sci., 116, 1095–1103, 2019.
Roblin, B., Ryan, M., Vreugdenhil, A., and Aherne, J.: Ambient Atmospheric Deposition of Anthropogenic Microfibers and Microplastics on the Western Periphery of Europe (Ireland), Environ. Sci. Technol., 54, 11100–11108, 2020.
Sander, M., Costa, E. S., Balbão, T. C., Carneiro, A. P. B., and dos Santos, C. R.: Debris recorded in ice free areas of an Antarctic Specially Managed Area (ASMA): Admiralty Bay, King George Island, Antarctic Peninsula, Neotrop. Biol. Conserv., 4, 36–39, 2009.
Sfriso, A. A., Tomio, Y., Rosso, B., Gambaro, A., Sfriso, A., Corami, F., Rastelli, E., Corinaldesi, C., Mistri, M., and Munari, C.: Microplastic accumulation in benthic invertebrates in Terra Nova Bay (Ross Sea, Antarctica), Environ. Int., 137, 105587, https://doi.org/10.1016/j.envint.2020.105587, 2020.
Shepherd, A., Fricker, H. A., and Farrell, S. L.: Trends and connections across the Antarctic cryosphere, Nature, 558, 223–232, 2018.
Simoes, C. L., Rosa, K. K. D., Czapela, F. F., Vieira, R., and Simoes, J. C.: Collins Glacier retreat process and regional climatic variations, King George Island, Antarctica, Geogr. Rev., 105, 462–471, 2015.
Suaria, G., Perold, V., Lee, J. R., Lebouard, F., Aliani, S., and Ryan, P. G.: Floating macro-and microplastics around the Southern Ocean: Results from the Antarctic Circumnavigation Expedition, Environ. Int., 136, 105494, https://doi.org/10.1016/j.envint.2020.105494, 2020.
von Friesen, L. W., Granberg, M. E., Pavlova, O., Magnusson, K., Hassellöv, M., and Gabrielsen, G. W.: Summer sea ice melt and wastewater are important local sources of microlitter to Svalbard waters, Environ. Int., 139, 105511, https://doi.org/10.1016/j.envint.2020.105511, 2020.
Wright, S., Ulke, J., Font, A., Chan, K., and Kelly, F.: Atmospheric microplastic deposition in an urban environment and an evaluation of transport, Environ. Int., 136, 105411, https://doi.org/10.1016/j.envint.2019.105411, 2020.
Zhang, M., Haward, M., and McGee, J.: Marine plastic pollution in the polar south: Responses from Antarctic Treaty System, Polar Rec., 56, https://doi.org/10.1017/S0032247420000388, 2020.