the Creative Commons Attribution 4.0 License.
the Creative Commons Attribution 4.0 License.
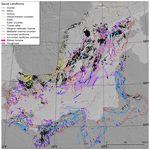
Reconstructing dynamics of the Baltic Ice Stream Complex during deglaciation of the Last Scandinavian Ice Sheet
Jakub Z. Kalita
Christiaan R. Diemont
Stephen J. Livingstone
Chris D. Clark
Martin Margold
Landforms left behind by the last Scandinavian Ice Sheet (SIS) offer an opportunity to investigate controls governing ice sheet dynamics. Terrestrial sectors of the ice sheet have received considerable attention from landform and stratigraphic investigations. In contrast, despite its geographical importance, the Baltic Sea remains poorly constrained due to limitations in bathymetric data. Both ice-sheet-scale investigations and regional studies at the southern periphery of the SIS have considered the Baltic depression to be a preferential route for ice flux towards the southern ice margin throughout the last glaciation. During the deglaciation the Baltic depression hosted the extensive Baltic Ice Lake, which likely exerted a considerable control on ice dynamics. Here we investigate the Baltic depression using newly available bathymetric data and peripheral topographic data. These data reveal an extensive landform suite stretching from Denmark in the west to Estonia in the east and from the southern European coast to the Åland Sea, comprising an area of 0.3 million km2. We use these landforms to reconstruct aspects of the ice dynamic history of the Baltic sector of the ice sheet. Landform evidence indicates a complex retreat pattern that changes from lobate ice margins with splaying lineations to parallel mega-scale glacial lineations (MSGLs) in the deeper depressions of the Baltic Basin. Ice margin still-stands on underlying geological structures indicate the likely importance of pinning points during deglaciation, resulting in a stepped retreat signal. Over the span of the study area we identify broad changes in the ice flow direction, ranging from SE–NW to N–S and then to NW–SE. MSGLs reveal distinct corridors of fast ice flow (ice streams) with widths of 30 km and up to 95 km in places, rather than the often-interpreted Baltic-wide (300 km) accelerated ice flow zone. These smaller ice streams are interpreted as having operated close behind the ice margin during late stages of deglaciation. Where previous ice-sheet-scale investigations inferred a single ice source, our mapping identifies flow and ice margin geometries from both Swedish and northern Bothnian sources. We anticipate that our landform mapping and interpretations may be used as a framework for more detailed empirical studies by identifying targets to acquire high-resolution bathymetry and sediment cores and also for comparison with numerical ice sheet modelling.
- Article
(15446 KB) - Full-text XML
-
Supplement
(4660 KB) - BibTeX
- EndNote
The Baltic depression (Fig. 1), currently occupied by the Baltic Sea, was a defining feature of the bed of the Scandinavian Ice Sheet (SIS), likely exerting considerable control on the direction of the ice flow and position of ice margins during the last glaciation (Holmlund and Fastook, 1995; Patton et al., 2017, 2016; Stroeven et al., 2016). The depression has a depth of 200 to 300 m (Fig. 1d) and is positioned such that it might have steered ice evacuation from the main ice divide towards ice margins in the south. The topographic steering of ice flow may have been further enhanced by unconsolidated sediments previously accumulated in the depression, reducing the basal shear stress and promoting faster ice flow velocities compared to the surrounding bedrock shield areas (Amantov et al., 2011; Boulton and Jones, 1979; Boulton et al., 1985). Glacio-isostatic effects of ice loading increased the reverse-slope setting of the southern Baltic, impeding water evacuation from the proglacial zone (Larson et al., 2006; Uścinowicz, 2003). The ice margin retreating across the Baltic depression from its Last Glacial Maximum (LGM) position was thus water-terminating, impacting ice dynamics due to calving and sub-aqueous melting (e.g. Noormets and Flodén, 2002b, a; Uścinowicz, 1999). Ice dynamics and changes in ice flow direction were also linked to ice divide shifting from the Scandinavian mountains to over the Bothnian Basin (Boulton et al., 1985; Kleman et al., 1997; Patton et al., 2016).
Despite the factors outlined above that might have influenced ice geometry and dynamics, much of the glacial geomorphological record within the Baltic depression remains undocumented because it is obscured by the modern-day Baltic Sea. There was no submarine evidence available at the time Boulton et al. (2001) reconstructed the ice stream pattern along the Baltic (Fig. 2); the aim of this paper is to seek information about the footprint of the ice stream and its likely width. We use a suite of newly available bathymetric and coastal datasets to map the glacial geomorphology across a ∼ 310 000 km2 region, encompassing the current Baltic Sea floor and adjoining coastal areas (Fig. 1b). We present a consistent sector-wide glacial landform map of the Baltic depression and use these data to reconstruct past ice flow geometries and major ice margin positions. Specifically, we use the mapped landform record to address the following questions.
-
As has frequently been suggested from empirical and modelling investigations (Boulton et al., 1985; Patton et al., 2017, 2016; Punkari, 1997; Stephan, 2001), does the landform record support a depression-wide (300 km) Baltic Ice Stream during the LGM?
-
What was the character of ice margin retreat through the Baltic depression? Is there evidence of water-terminating calving ice fronts or lobate terrestrial-style margins?
-
To what extent did topographic pinning points arising from geological structures or lithological contrasts influence ice margin retreat?
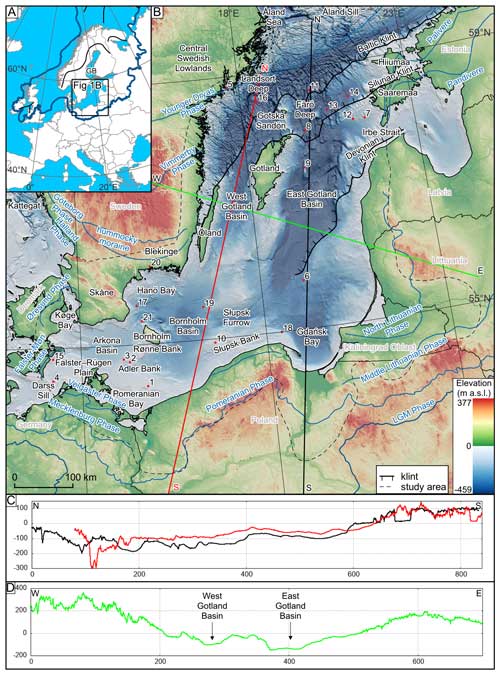
Figure 1(a) The Last Glacial Maximum extent over Europe (thick blue line; Svendsen et al., 2004). GB indicates the Gulf of Bothnia, located north of the study area. Black line indicates ice divides around the LGM (after Kleman et al., 2008; Patton et al., 2016). The base map is from EuroGeographics and the UN FAO. (b) The study area, which covers the Baltic Sea and the nearby coastal zone. Major phases are drawn in blue, with their names indicated. The Baltic Klint constitutes a margin between crystalline bedrock (to the north) and Ordovician sedimentary rocks (to the south). The Silurian Klint separates Ordovician (north) and Silurian rocks (south). Red dots and the associated numbers indicate key previous studies of offshore glacial landforms (see Table 1). The DEM is based on EU-DEM and EMODnet (see Table 2). (c) The N–S profiles (see the black and red lines in panel b) reveal a general upslope toward the south, which would have been even steeper during glaciation due to glacio-isostatic loading, and cuesta-like topography associated with geological steps at the Baltic Sea bottom. (d) The NW–SE profile (see the green line in panel b) depicts the ∼ 400 km wide onshore and offshore depressions divided by Gotland into two troughs: eastern and western along the East and West Gotland basins respectively.
The effects of the Baltic depression on the dynamics of the Last SIS have been discussed since the late 19th century (Madsen, 1898; Zeise, 1889, after Stephan, 2001). This includes evidence of a major, up to 130°, switch in ice flow direction, from towards the south to the west and north-westward in the region of the southern Baltic and Denmark. The north-westward and westward flows into Denmark, after the LGM, have subsequently been termed the Young Baltic Advances (Holmström, 1904, in Glückert, 1974; Stephan, 2001) (Fig. 2, arrow B1). Subsequently, it was hypothesised that this change in ice flow direction in the southern sector of the ice sheet was due to an eastward shift of the main ice divide (Ahlmann et al., 1942; Eissmann, 1967; Enquist, 1918; Ljunger, 1943; Woldstedt and Duphorn, 1974). An alternative explanation for the flow directional changes was that the upland topography behind the German and Polish coasts caused ice to be redirected westward (e.g. Gripp, 1981, after Stephan, 2001). Such behaviour could be a consequence of increasing topographic dependence during deglaciation (Kjær et al., 2003). Lithostratigraphy and striae analysis by Ringberg (1988) indicated that reduced ice supply over Sweden caused the Baltic Ice Stream to turn westwards into Denmark when the ice sheet was retreating from its maximum extent (Figs. 2 and B1). The westward ice flow redirection was also modelled by Boulton et al. (1985). North-westward flow into Skåne and Denmark has also been suggested for the period prior to the maximum ice sheet extent (termed the Old Baltic Advance, Ringberg, 1988), based on the lithostratigraphy of till deposited in Denmark and from striae orientations.
Boulton et al. (2001) conducted one of the earliest satellite-driven mapping campaigns of glacial lineations at the ice sheet scale, resulting in possible ice stream configurations during deglaciation (Fig. 2). Subsequent landform investigations using higher-resolution data identified potential palaeo-ice streams in the marine and terrestrial record within and surrounding the Baltic Sea, based on the identification of mega-scale glacial lineations (MSGLs) (Greenwood et al., 2016; Jögensen and Piotrowski, 2003; Kalm, 2012; Szuman et al., 2021; Greenwood et al., 2024). These ice stream imprints commonly conform to local topographic depressions, indicating that topography played a role in flow geometry, at least regionally, during ice retreat.
As our observational record of modern ice sheet dynamics expanded, so did the idea that the Baltic depression facilitated an ice stream that operated throughout the Last Glacial (Holmlund and Fastook, 1995; Patton et al., 2017, 2016; Stroeven et al., 2016). Numerical ice sheet modelling efforts focused on the SIS, typically input basal shear stress values based on weak substrates and an assumed existence of ice streams here (Holmlund and Fastook, 1995; Patton et al., 2017, 2016), resulting in synchronous, Baltic-wide ice streaming in the low-shear-stress regions. It therefore remains difficult to determine whether the Baltic ice streams suggested by some of these earlier studies are realistic.
Sector-scale landform mapping has been carried out for the Bothnian Basin from high-resolution bathymetry data (Greenwood et al., 2015, 2016). These data revealed early Holocene ice streaming, documented by cross-cutting glacial lineations and an abundance of meltwater landforms and crevasse-squeeze ridges (e.g. Greenwood et al., 2016). In contrast, patchy bathymetric data have until very recently (see Greenwood et al., 2024, for an independent study of the same area) limited investigations in the Baltic depression to localised reconstructions and extrapolation from nearby terrestrial records (Boulton et al., 2001; Cuzzone et al., 2016; Kleman et al., 1997), mixed with inferences from glaciological intuitions and hunches (Fig. 1).
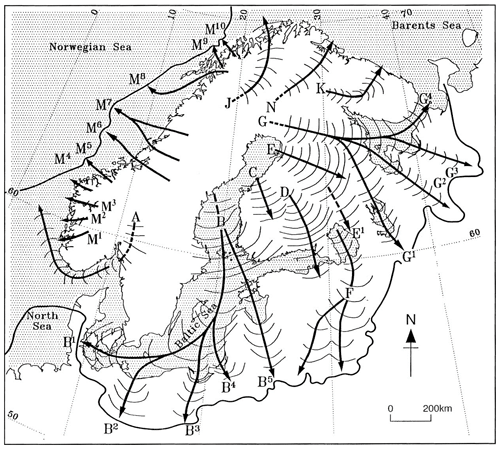
Figure 2An early depiction of time-transgressive ice stream trajectories with associated back-stepping ice margins after Boulton et al. (2001; reprinted from Quaternary Science Reviews, © (2001), with permission from Elsevier). The Baltic Sea depression is inferred to control the ice stream location, but note that this depiction does not imply a synchronous Baltic Ice Stream of this length (> 1000 km) branching into B1 to B4. Instead, it depicts numerous short-length (< 300 km) ice streams on land (B1 to B4) that, once back-stepped into the Baltic depression, eventually merged into a single ice stream. No submarine evidence was available at the time Boulton et al. (2001) drew the figure, and it was the purpose of this paper to seek information about the footprint of this ice stream and its likely width. The relative timing of the ice streams draining the Baltic depression has been established only partially, with Kjær et al. (2003) and Houmark-Nielsen and Kjær (2003) noting that B1 (called the Young Baltic Advances) came after the B2 lobe into Germany and Poland.
The bathymetry of the Baltic Sea, with its prominent N–S- and E–W-trending depressions, has been attributed to the geological structures of the region and its differential erosion by Pleistocene ice sheets (Hall and Van Boeckel, 2020; Poprawa et al., 1999). The north-western Baltic Basin has a crystalline Proterozoic substrate typical of the Scandinavian Shield and, where the sediment cover is limited, the bedrock structure is clearly visible in the bathymetric data (Fig. 1b). Stratigraphic and seismic surveys have indicated that the sets of prominent NE–SW-oriented ridges (“klints”) between Estonia and Sweden correspond to lithological transitions between Cambrian, Ordovician, and Silurian rocks, controlling erodibility and generating stepped morphologies (Fig. 1c) (Tuuling and Flodén, 2016). The south-eastern Baltic Basin is dominated by more readily erodible lithologies with Paleogenic and Neogenic sandstones and mudstone sequences. Sediment thickness and composition vary throughout the Baltic, with the northern part having patchier cover between 20 and 30 m in thickness, while sediment cover further south is more extensive, with thicknesses of up to 60 m (Björck et al., 1990; Noormets and Flodén, 2002b, a; Uścinowicz, 1999). The surficial deposits comprise glaciogenic sands and lacustrine sediments as well as marine strata (Sviridov and Emelyanov, 2000). Zones of unconsolidated sediment accumulation occur throughout the whole Baltic Sea. Those in the northern sector are composed of glaciofluvial material and were interpreted as subglacial outwash deposits and ice margin grounding line deposits (Noormets and Flodén, 2002b). Accumulations of unconsolidated glaciofluvial and glacial sediments particularly correlate with the positions of sills and banks (Kramarska, 1998; Lemke and Kuijpers, 1995; Obst et al., 2017; Uścinowicz, 1999; Noormets and Flodén, 2002a).
In this study, we map landforms in submarine and peripheral coastal regions (Fig. 1b) of the present-day Baltic Sea. The offshore area coincides with the so-called Baltic Proper, an area extending from the Åland Sill in the north to the Darss Sill in the south-west and the islands of Hiiumaa and Saaremaa in the east. Our motivation for mapping the coastal periphery of the Baltic is twofold.
-
It eases integration with the much-studied onshore landform record.
-
To provide a high-resolution verification for ice flow patterns and ice margin retreat patterns mapped from the lower-quality and lower-resolution bathymetric dataset.
Bathymetric data varied substantially in resolution and quality across offshore regions. We used the Digital Bathymetry Model of the European Marine Observation and Data Network versions 2018 and 2022 (EMODnet Bathymetry Consortium, 2022). Its source data are a conglomerate of data sets with resolutions typically between 20 and 50 m but with some regions of 200 to 500 m resolution (Fig. 3) (Jakobsson et al., 2019). Confident identification of glacial landforms was not possible in the low-resolution regions. Artifacts are common in the dataset (see e.g. Fig. 3b) and were cross-checked with hydro-acoustic and seismic data (Table 1) where available. High-resolution (1–5 m) digital elevation models (DEMs) were used to map the peripheral terrestrial regions (Table 2), with the exception of the Kaliningrad region, where a 25 m resolution DEM was used. Mapping was carried out manually using the open-source Quantum Geographic Information System (QGIS). Semi-transparent hillshades with numerous azimuth angles to limit visualisation bias (Smith and Clark, 2005) were superimposed onto the DEM. Vertical exaggerations between 1 and 40 assisted in the identification of low-amplitude landforms, with cross-profiles and colour palette manipulation proving a powerful tool for verifying landforms, especially in regions of low resolution and quality of data. When possible, geological maps (Table 3) were used to support our interpretations, although the resolution typically exceeds the size of the landforms and only provides an indication of surficial sediments. Our approach was focused on the geomorphological expression of landforms not on their surface geology. For example, if a ridge met the characteristics of a moraine ridge (fan-shaped, perpendicular to the ice flow direction and, in ideal situations, associated with other landforms), it was judged as such even if it was covered by e.g. marine sands.
Glacial lineations were mapped along the crest line using polylines and included features in bedrock and unconsolidated sediments such as MSGLs, drumlins, crag-and-tails, and grooves. Given their linear nature and similarity to some known artifacts in low-resolution data, lineations were sub-divided into different confidence levels (“glacial lineations” and “uncertain glacial lineations”). Ribbed moraines and regions of iceberg plough marks were mapped as polygons. We also mapped ice-contact landforms as polylines along their highest-elevation area and with two confidence levels. Ice-contact landforms encompass moraines, grounding zone wedges (GZWs), and ice-contact fans. All are oriented perpendicular to the ice flow direction. Moraines have the most prominent fan-shaped ridge, with a roughly symmetrical cross-profile. In contrast, GZWs have a steep distal slope and a gentle proximal slope with a sinusoidal pattern of ridges that often occur in swarms. Ice-contact fans have steep proximal and gentle distal slopes. Eskers were mapped along the crest line and grouped into two confidence levels. Meltwater channels were mapped by polylines along their lateral margins and classified into tunnel valleys, marginal meltwater channels, and uncertain meltwater channels. Tunnel valleys were distinguished based on their orientation parallel to glacier flow. They are often associated with eskers, terminate near former ice margins, and have undulating long profiles (Kehew et al., 2012). In some offshore localities associated with the low-resolution DEM or unclear situation (e.g. a lack of eskers), tunnel valleys were mapped as uncertain. Marginal meltwater channels often form a swarm of parallel channels that document the positions of former glacier margins (Margold et al., 2013). We did not map meltwater channels along the Swedish coast from Blekinge towards the Central Swedish Lowlands and the northern Baltic, where the hard bedrock geology contains numerous fissure valleys with complicated patterns, with only some occupied by eskers.
The mapped glacial lineations formed the basis for developing flow sets (Boulton and Clark, 1990; Clark, 1990; Greenwood and Clark, 2009). A flow set comprises a coherent set of morphologically and directionally associated lineations, interpreted as representing a discrete ice flow event. Low-resolution data in regions such as the eastern part of East Gotland Basin and large parts of the southern Baltic (i.e. Arkona and Bornholm basins, Słupsk Furrow, and Gdańsk Bay; Figs. 1b, 3) resulted in gaps between regions of higher-density mapping. However, it was often possible to detect larger features in these regions by investigating multiple variants of hillshade angles, z factors, colour palettes, and terrain profiles and comparing them with available studies (Table 1).
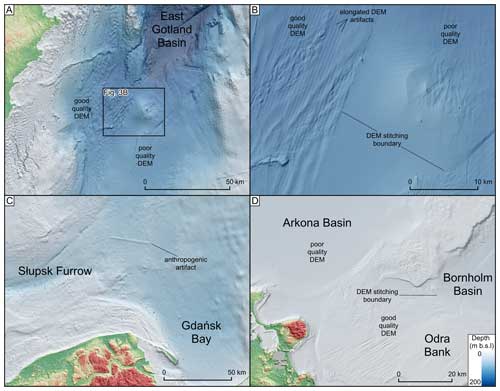
Figure 3Examples of DEM artifacts and high- and low-resolution bathymetric data. The DEM is based on EMODnet (see Table 2). (a) Higher-resolution bathymetric data for the western part of the East Gotland Basin enabled landform recognition, whereas the low-resolution data for the eastern part made it difficult to identify glacial landforms. The onshore DEM is based on the EU-DEM (see Table 2). (b) Examples of DEM artifacts in low- and high-resolution data, especially visible along the DEM stitching boundary. Note the difficulty in identifying landforms in the low-quality data region. (c) Low-resolution DEM for the southern Baltic, with landforms not distinguishable in Gdańsk Bay but slightly more visible along the Słupsk Furrow. The onshore DEM was provided by GUGIK (see Table 2). (d) The contrasting data quality for the high-resolution DEM of Odra Bank vs. the low-resolution DEM along the Arkona and Bornholm basins. The onshore DEM was provided by © GeoBasis-DE/M-V 2020 (see Table 2).
About 25 000 features were mapped within the study area (Fig. 4). Most of the regions with higher-resolution data (both marine and terrestrial) display glacial landforms. The offshore features constitute 25 % of the total object population. The highest density of landforms is found along the Swedish, German, Polish, and Lithuanian coasts and in the vicinity of the area between the islands of Öland and Gotland. The lowest density of landforms is along the central and southern Baltic Sea. However, the few landforms found here are of considerable sizes. Glacial lineations comprise ca. 15 000 of the mapped features, with eskers numbering ca. 5200. The majority (in terms of percentage) of the lineations and eskers were mapped in the coastal regions. Burial of landforms in marine environments has been identified before (Flodén et al., 1997; Kirkham et al., 2022), and so our mapping is likely an incomplete morphological record in parts of the Baltic depression.
4.1 Streamlined bedforms
A variety of glacial streamlined bedforms, including MSGLs, drumlins, crag-and-tails, and grooves, are mapped in the study region (Fig. 5), consistent with mapping from other studies in the region (Noormets and Flodén 2002a; Schäfer et al., 2021). Streamlined bedforms are widely distributed and found in regions of soft sedimentary rocks or areas of thin drift over predominantly bedrock-exposed regions. Glacial lineations occur throughout the Baltic Sea to a depth of at least 260 m b.s.l. Almost 75 % of the glacial lineations are shorter than 1200 m. They cluster in the terrestrial parts of the Baltic Shield and in the southern sector of the SIS in Poland (Dowling et al., 2015; Hermanowski et al., 2019). MSGLs are found in the deeper depressions and extend in length up to 80 km. Highly parallel MSGLs start abruptly at the geological transition of the Baltic Klint, between the crystalline shield in the northern Baltic and sedimentary rocks to the south (see also Tuuling and Flodén, 2001, 2016). The densest concentrations of glacial lineations were mapped in Landsort Deep (Figs. 4 and 5g), where they are oriented N–S and NW–SE, with the latter dominant. They occupy pre-Quaternary sandstones, in contrast to the surrounding hard bedrock to the north, east, and west (All et al., 2006).
A swarm of 138 grooves occurs between the klints. The grooves are inter-mixed with drumlins and exhibit high parallel conformity with them (Fig. 5h). The grooves are straight, with an average length of ca. 1300 m. In the northern Baltic, the MSGLs are highly parallel, and their density and length increase towards the central parts of the two troughs located either side of Gotland. Sparse eskers and meltwater channels with lengths up to 95 km also occur in the troughs. Glacial lineations, up to 5000 m long, in the terrestrial south form local fields typically restricted to drumlinised belts located close to arcuate moraines and marginal meltwater channels. However, these sets often comprise fewer than 30 lineations.
A concentration of offshore glacial lineations occurs in the southern Baltic in Pomeranian Bay (Fig. 7d). This area displays highly elongated lineations with an average length of 4450 m and widths of 200 to 500 m. The lineations are interpreted as glacial in origin but with low confidence given their waviness and overlapping nature. They could also be formed by bottom currents or a mixture of glacial and non-glacial processes (i.e. exposed by erosional activity of bottom currents). Klingberg and Larsson (2017) identified lineations eroded by currents west of Öland. However, they are clear erosional landforms with an anastomosing pattern of grooves and irregular shapes (changeable width and general geometry) of “positive” sections. MSGLs in Pomeranian Bay (Fig. 7d) have more or less the same width along the whole profile and a regular shape. They have a potentially erosional and depositional origin as the ridges clearly overlap each other from different azimuths, and they represent positive and negative landforms. In the vicinity (point 1 in Fig. 1b) there are ridges composed of glacial till with lineation-like cross profiles (Kramarska, 1998) buried in postglacial sands. Further detailed studies are required to confidently interpret the landforms in Pomeranian Bay.
Cross-cutting of streamlined bedforms is common in the study area, especially in Skåne, Germany, Poland, Öland, and Gotland as well as offshore in the West and East Gotland basins and Pomeranian Bay. Typically, more delicate and shorter glacial lineations overprint more prominent ones. In contrast, replacement of cross-cutting with overlapping (with the same orientation) relationships is identified in the eastern part of the study area.
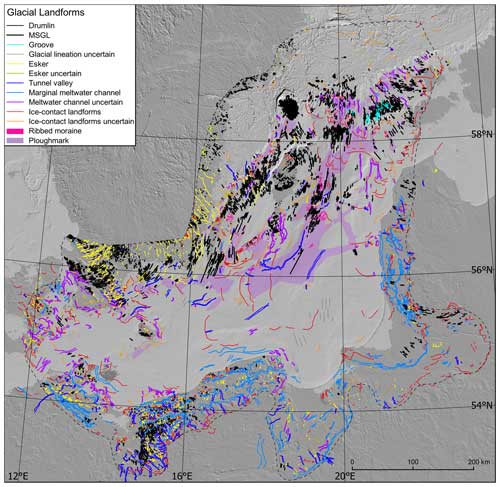
Figure 4Landforms mapped in this study. A high-resolution version can be downloaded from the Supplement (Fig. S1). Light and dark grey indicate offshore and onshore areas respectively. Notice the small number of landforms in the offshore northern part of the study area floored by hard bedrock. The DEM is based on EU-DEM and EMODnet (see Table 2).
4.2 Ribbed moraines
Ribbed moraines are identified in the study area in five offshore localities and one onshore locality (e.g. Fig. 5b, i) and typically exhibit a spatial transition downstream into glacial lineations. Ribs are often associated with and oriented perpendicularly to topographic highs and obstacles (Fig. 5b). In one locality, in the East Gotland Basin, glacial lineations are superimposed onto ribbed moraines.
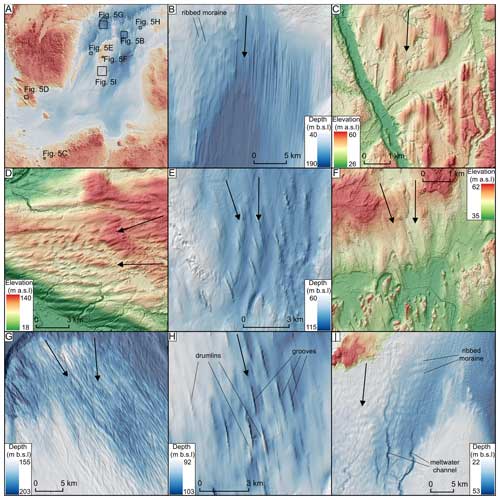
Figure 5Examples of glacial lineations, grooves, and ribbed moraines found in the study area. Ice flow direction is indicated by black arrows. The DEMs are based on EU-DEM and EMODnet if not stated otherwise (see Table 2). (a) Locations of landform examples. (b) MSGLs along Fårö deep and ribbed moraines to the north-west. (c) Drumlins on the Polish coast. The DEM is provided by GUGIK (see Table 2). (d) MSGLs in Skåne, Sweden, oriented E–W and cross-cut by NE–SW-oriented drumlins. The DEM is provided by © Lantmäteriet. (e) Cross-cutting glacial lineations in the West Gotland Basin. (f) Crag-and-tails in southern Gotland, overprinting larger N–S-oriented MSGLs. Note the moats (scour marks) around the stoss sides of the crag-and-tails. The DEM is provided by © Lantmäteriet. (g) Cross-cutting glacial lineations in the vicinity of Landsort Deep. Note that the NW–SE-trending lineations towards the East Gotland Basin are more elongated and better developed than the N–S-trending lineations towards the West Gotland Basin. This overlapping arrangement, seen here, is not common across the study area. (h) Glacial grooves identified in an area of basal till are overlain by lacustrine and marine deposits (see Noormets and Flodén, 2002a) on the Silurian basement, between klints. (i) Ridges south of Gotland are oriented perpendicularly to the inferred ice flow direction and are interpreted as ribbed moraine.
4.3 Flow sets and their relative age relationship
Figure 6 shows the 77 flow sets that were identified. The flow sets display a range of geometries with parallel lineation sets in deeper sections of the Baltic and splaying geometries found across much of the study area from the terrestrial southern margin to the regions further north in the vicinity of the klints. Flow-set orientations vary substantially in the study region, documenting ice flow directions to the east in the eastern Baltic and to the north-west in the western part of the study area, overprinting (e.g. Fig. 6b, c) on Gotland records 10 flow sets with orientations switching from N–S to NNE–SSW through to NE–SW, ENE–WSW, and NW–SE (Fig. 6c). Flow-set relative timing was distinguishable in several parts of the study area by superposition, cross-cutting, and smudging of landforms (Fig. 6). We suppose that such complex shifts in ice flow direction were likely common through the study area but are not always visible due to low-resolution data. Individual flow sets with no overprinting relationships (Fig. 6a) dominate in the eastern and northernmost parts of the study area.
4.4 Ice-contact and meltwater landforms
A range of ice-contact landforms were mapped (Fig. 7), including moraine ridges (e.g. Fig. 7b), ice-contact fans (e.g. Fig. 7e), and GZWs (Fig. 7c, f, i). The most prominent ice-contact landforms are found in the central Baltic, where they are up to 30 m high and 45 km long (Fig. 7e), with steep proximal and gentle distal slopes. The only information on their sedimentological composition comes from limited seismo-acoustic profiles, which indicate that landforms are composed of till and glaciofluvial sediments (Uścinowicz, 1999). The cross-profiles combined with the shallow water in the region during deglaciation indicate these are not grounding zone wedges but ice-contact fans. An ice-contact fan in the vicinity of Słupsk Bank was interpreted by Uścinowicz (1999) as a glaciofluvial delta. The broad, up to 37 km long, 50 km wide, 34 m high, and only 13 m b.s.l. ice-contact landform east of Öland (Fig. 7e, i) has a steeper distal and gentle proximal slope with moraines superimposed on it. The size and shape resemble a grounding zone wedge (see Batchelor and Dowdeswell, 2015). Other ice-contact landforms to the north (along the klints) typically have steep distal slopes and wider and lower-angled proximal slopes (Fig. 7c, f). They are composed of thick glaciofluvial sediments (up to 55 m; Noormets and Flodén, 2002a) and are up to 40 km long. These ice-contact landforms, along the klints, are interpreted as grounding zone wedges (see Batchelor and Dowdeswell, 2015), although their limited amplitudes (from only 5 m) and local transitions to moraines may indicate that the free space beneath the ice shelf was shallow and floating occurred locally. Locally, moraines are visible on top of and next to GZWs (e.g. Fig. 7c, f, i). The two ice-contact landforms at Pomeranian Bay and the Darss Sill–Falster-Rügen Plain (Figs. 7d and 9b) are almost flat and 40 to 70 km long, composed mainly of glacial tills and Pleistocene sand and silt and are covered by fine glaciolacustrine and marine sediments (see Lemke et al., 1994; Lampe et al., 2011; Kramarska, 1998) that resemble flat-topped ice-contact fans. Moraines represent a broad spectrum of sizes, from wide (up to 3500 m) and high (up to ca. 20 m) to narrow (less than 300 m), steep, and up to 4 m high (Fig. 7b, c, f). Their internal composition has been recorded in the southern Baltic (Fig. 9b) and in the area near the Darss Sill–Falster-Rügen Plain, where Jensen (1993) identified a series of small thrusted moraines composed of stratified clays overlain by glacial diamicton. A series of closely spaced moraines are also identified locally along Irbe Strait (Fig. 7c) and east of Öland (Fig. 7i) with 3–5 m amplitude and 300–400 m width.
Cross-cutting and overprinting of moraines and ice-contact fans occur in the vicinity of the Danish islands, eastern Germany, and Słupsk Bank (Fig. 7d) in the central Baltic. Cross-cutting relationships such as those in the central Baltic record changes in ice flow geometry during retreat. Here a distinct N–NW, NE–N, and E–W flow geometry can be inferred (Fig. 7e), with ice-contact landforms associated with the NE–N ice flow direction being the youngest.
Single- or multiple-crested eskers are common (n>3000) in the west and south-west of the study area (e.g. Figs. 7g and 9e) but are almost completely absent in the east (East Gotland Basin and eastern Baltic coast). Most of the eskers identified in this study occur onshore, with only 2 % of the total population located offshore. The longest and most complex eskers occur in southern Sweden, where they form dendritic networks (Dewald et al., 2022; Stroeven et al., 2016), and offshore from here, where eskers are up to ca. 45 km long. The offshore eskers located in the vicinity of the Swedish coast conform to the splaying pattern of the inland esker networks. Another group of SSW-oriented single-ridged offshore eskers, up to 45 km long, aligns with the streamlined bedforms in the deepest parts of the Baltic Sea. The two esker groups of opposing orientations are divided by the island of Gotland. Along the klints, Noormets and Flóden (2002a) mapped several broad (up to 12 km wide) eskers. However, we interpreted and mapped them as ice-contact landforms on the basis of the fan-shaped morphologies along at least 90 km (see the margin indicated by the black line starting at Gotska Sandön (GS) toward the east in Fig. 8) and their similarity to the moraines and GZWs presented in Fig. 7f. Eskers are not present in offshore regions of the southern Baltic and are common but short (< 10 km in length) in the adjoining coastal areas of northern Germany and Poland (Frydrych, 2022). With the given DEM resolution, we did not detect any eskers at Adler Bank (Feldens et al., 2013). Here we only detected ridges oriented transverse to the ice flow direction that mimic small, 2–3 m high, recessional moraines (Fig. 4). The general distribution of better-developed esker networks on the shield compared to regions of sedimentary lithologies is consistent with observations from the former Laurentide Ice Sheet in North America (Clark and Walder, 1994). We speculate that such small landforms might not be distinguishable in the poor-quality DEM available for the offshore areas or that eskers could be buried by postglacial deposits (Uścinowicz, 1999).
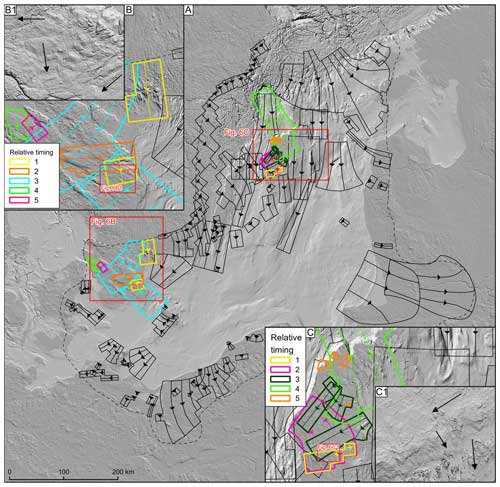
Figure 6(a) The flow sets defined for the study area (dashed line). Close-ups of flow sets in the area of the islands of (b) Skåne and (c) Gotland. Colours indicate their relative age based on overprinting relationships. Higher numbers in panels (b) and (c) are younger. The relative timing of green (4) and pink (5) flow sets in panel (b) is uncertain. The DEM is based on EU-DEM and EMODnet (see Table 2).
Ice margin meltwater channels are often associated with the lobate shape of the moraines located in their vicinity. They vary in size, from long (exceeding 100 km sections), wide (up to 5 km), and well-preserved channels (in e.g. Germany, Poland, or Kaliningrad) to short and fragmented (10–100 m wide) channel segments (Fig. 7h). The ice margin meltwater channels along the Kaliningrad, Poland, and German coasts form semi-continuous drainage systems almost 400 km long. Offshore meltwater channels are generally restricted to the southern part of the study area; the widest examples here are up to 7 km in cross section (along the Polish coast).
Tunnel valleys are identified both onshore and offshore (Figs. 7e, g, 9b, e), some up to 90 km in length. Tunnel valleys typically do not exceed 2 km in width, consistent with other examples from Scandinavia (see Jørgensen and Sandersen, 2006). They often occupy geological structures along the Swedish coast and the klints, whereas in the southern sector they occur in unconsolidated sediments. Terrestrial examples are often associated with eskers, but only nine such associations are identified offshore.
Much of the sea bottom in the central and northern sectors of the Baltic Sea is covered with iceberg plough marks (Figs. 4 and 7f). They have an identifiable U- to V-shaped cross-profile with thalwegs that are typically curvilinear to straight. The plough marks rarely exceed a depth of 2 m, and their length varies between 45 m and 20 km, consistent with other studies on plough marks in the region (Dorokhov et al., 2018; Karpin et al., 2021). However, in some areas the resolution of the DEM hampered plough-mark detection, e.g. the ∼ 220 km2 field detected by Dorokhov et al. (2018) in the central Baltic in the vicinity of our large, ∼ 16 970 km2 field. Orientations vary from perpendicular to the inferred ice margins to chaotic with plough marks cross-cutting each other. No plough marks are identified in the southernmost part of the study area (Fig. 4), down-ice from the Adler and Odra banks, either because of the low DEM resolution or the shallow water depth.
4.5 Ice margin positions
To reconstruct the character of the ice margins and the pattern of deglaciation, we used the suite of mapped landforms and our glaciological understanding of how they formed to infer major ice margin retreat geometries (Fig. 8). The margin configuration along the terrestrial southern sector spanning Denmark, Germany, Poland, the Kaliningrad region, and Lithuania comprised a series of lobes as evidenced by meltwater channels, eskers, and moraines. The spacing between the identified ice margin positions varies, with the southern sector generally having a closer spacing of about 10 to 40 km. Ice retreat down a reverse slope is reflected in the presence of long (almost 400 km) ice margin channel systems that are well-preserved along the German, Polish, and Kaliningrad coasts. Offshore, ice margin positions are mainly identified from ice-contact landforms. Along the eastern Baltic, ice margin positions typically have a 40–80 km spacing, with 180 km between the central Baltic ice-contact landforms and the Devonian Klint (Fig. 1) and 120 km between the Silurian Klint and Åland Sill. In the western Baltic the spacing is similar, up to ca. 80 km, with a larger gap of ca. 100 km east of Öland. It is probable that uniform and better-quality data for the whole Baltic area would permit the detection of more closely spaced landforms.
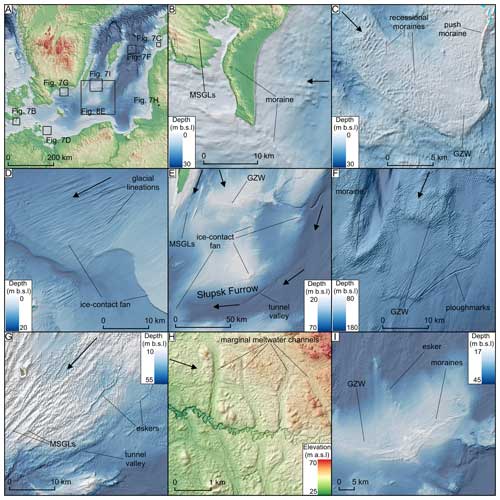
Figure 7Examples of ice margin landforms. Ice flow direction is indicated by black arrows. The DEMs are based on EU-DEM and EMODnet if not stated otherwise (see Table 2). (a) Locations of landform examples. (b) Example of moraine, onshore and offshore, in Denmark. The onshore DEM is provided by Styrelsen for dataforsyning og effektivisering (see Table 2). (c) Sequence of small, closely spaced moraines at Irbe Strait on the top of a grounding zone wedge with a push moraine visible to the east. (d) Ice-contact fan overprinted by enigmatic lineations, possibly of glacial origin, in the shallow waters of Pomeranian Bay. The sharp banks truncating the lineations are thought to form due to drainage of a local ice-dammed lake (see Uścinowicz, 1999). (e) The central Baltic, near the Słupsk Furrow, is occupied by the most prominent ice-contact landforms (ice-contact fans) in the study area. Tunnel valleys are oriented towards the westernmost ice-contact fan. East of Öland a grounding zone wedge is visible (see the close-up in panel (i)). (f) Glaciofluvial deposits down-ice from a pinning point (Noormets and Flodén, 2002b, a) comprising GZWs and moraine, covered by plough marks. (g) Eskers and an associated tunnel valley system in Hanö Bay, parallel to MSGLs. (h) Examples of marginal meltwater channels in Latvia. The DEM is provided by Latvijas Ìeotelpiskās informācijas aìentūra (see Table 2). (i) The GZW east of Öland with a series of small moraines and an esker in the central part. Notice that the lobe is in contact with the ice-contact fan to the east; for a zoom-out, look at panel (e).
In some offshore localities, the ice margin separates into two lobes, with a clear interlobate (suture) zone (Fig. 8). In Hanö Bay and east of Öland, the ice margin forms two 55–80 km wide lobes, each with eskers oriented perpendicular to the ice-contact landforms (e.g. Figs. 7i, 8, and 9e). In the central part of the study area, separate arcuate ice margin positions are reconstructed east and west of the SW–NE-oriented ridge of Gotland and its offshore southern continuation (Fig. 8). The marginal pattern becomes more lobate to the north of the study area, with a clear interlobate (suture) zone along GS (Fig. 8).
Our landform mapping of the previously mostly unexplored Baltic Basin (0.3 million km2) (although see also Greenwood et al., 2024) provides new information on the dynamics of the southern margin of the SIS during the last deglaciation. While our paper was in the reviewing stage, a similar study on glacial landforms of the Baltic was published (Greenwood et al., 2024), and we suggest that interested readers should consult both sources, noting that they were entirely independent investigations. Our study presents the glacial geomorphological mapping for the whole Baltic Proper and the adjacent coastal zone but is of a lower resolution for offshore areas, whereas Greenwood et al. (2024) is based on available patches of higher-resolution data in the Baltic but does not include coastal zones and presents fewer details for the central and southern Baltic. Reassuringly, there is a large amount of similarity between the two studies, especially for the general offshore glacial lineation pattern, eskers, and plough-mark positions. The largest differences in mapping are concentrated on Gotland and south of Gotland, where the higher-resolution data used by Greenwood et al. (2024) allowed for detection of more ribbed moraines and eskers.
Our mapping helps to fill the gap in reconstructions of the SIS over the Baltic Sea (e.g. Boulton et al., 2001; Stroeven et al., 2016; Tylmann and Uścinowicz, 2022) that previously had to rely on inferences and extrapolations from the adjacent terrestrial evidence. Below we use the reconstructed flow sets and ice margin positions to address the research questions raised in the Introduction.
5.1 Was there a Baltic-wide ice stream?
The existence of a large Baltic-wide (300 km) ice stream at the local LGM, feeding ice to the southern margin and westwards to Denmark (e.g. Holmlund and Fastook, 1995; see Fig. 2; Stephan, 2001), is largely based on glaciological inferences stemming from the broad-scale morphology of the ice sheet bed and its lithological properties rather than on a landform-defined footprint of the ice stream. We do not identify a landform “footprint” of simultaneous fast ice flow spanning the Baltic depression; the landforms in the central Baltic appear to record more fragmented corridors of fast ice flow consistent with later stages of deglaciation and smaller ice stream widths (typically 30 to 60 km, up to 95 km; Figs. 6 and 9c). Such widths are more typical of ice streams found elsewhere (e.g. Gandy et al., 2019; Livingstone et al., 2012; Margold et al., 2015; Stokes, 2018). This is indicated by the presence of distinct flow sets located in the vicinity of Gotland (Fig. 6) and multiple lobes indicated by the marginal retreat geometry in the central Baltic (Fig. 8). We interpret the flow sets confined to the depressions and flanking slopes either side of Gotland (Figs. 6 and 9c) and consisting of highly parallel MSGLs (Fig. 4) as the trunks of a number of narrow (30–60 km) palaeo-ice streams that operated during deglaciation behind a back-stepping ice margin. Whilst ice must have traversed the Baltic earlier to reach the maximum southern extent of the last SIS, as evidenced by the provenance of glacigenic sediments in the southern study area (e.g. Kjær et al., 2003; Woźniak and Czubla, 2015), we do not find any landform evidence in the Baltic depression that we could interpret as originating from this stage. Landforms from the maximum phase of glaciation may have been erased, buried, or not yet found.
Some ice sheet modelling experiments (e.g. Patton et al., 2017, 2016) reproduce a Baltic-wide area of fast flow (an ice stream) through much of the growth and decay of the ice sheet. We suggest that this fast flow zone is a response to the prescription of a soft substrate in the Baltic, in contrast to the hard-bedded surroundings (Patton et al., 2016, 2017). Modern observations of e.g. the Siple Coast ice streams in Antarctica (Catania et al., 2012) and numerical modelling experiments (Fowler and Johnson, 1996; Hindmarsh, 2009; Payne, 1998) suggest that narrow corridors of fast ice flow can exist even in soft-bedded regions of little relief; this is, in fact, what Bennett (2003) defined as “pure ice streams”. We therefore suggest that some width-limiting processes on ice stream formation have yet to be sufficiently added to numerical ice sheet models. Gandy et al. (2019) made progress with such work for the British–Irish Ice Sheet, where they explored the ingredients required in their modelling experiments to simulate ice streams in the correct places and with appropriate spacing and widths. They used the BISICLES higher-order model, which as well as having variable cell-size resolution, which helps with apportioning ice steaming, also incorporates approximations of membrane stresses which are necessary for yielding accurate ice streams.
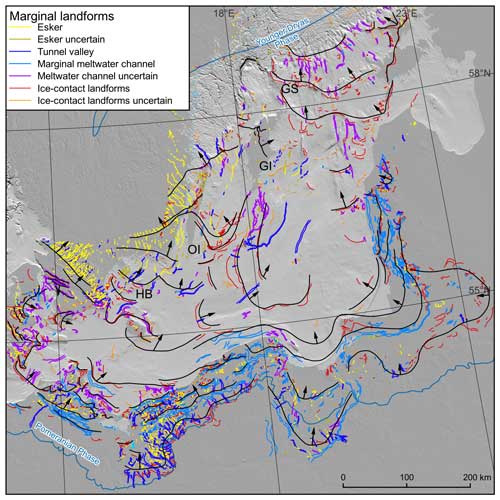
Figure 8Broad overview of possible ice margin retreat geometries (black lines with arrows showing the direction of retreat) inferred from the new mapping of landforms. Gaps in the bathymetric data and the known complexity of flow sets and flow-switching through time mean that the ice sheet retreat was likely more complex than illustrated here. Note the highly lobate nature of the ice margin to the south-west and south-east and some offshore examples of inter-lobate (suture) zones where the ice margin separates into two lobes: at Hanö Bay (HB) and over Öland (OI) and along Gotland (GI) and Gotska Sandön (GS). The DEM is based on EU-DEM and EMODnet (see Table 2).
5.2 What was the character of ice margin retreat though the Baltic depression?
Rather than congruent Baltic-wide ice streaming, flow sets can generally be incorporated into a time-transgressive deglacial retreat sequence that in some cases conforms to the pattern of reconstructed ice margins (Fig. 8). We find that the ice margin geometry changes significantly during retreat through the Baltic (Fig. 9b, c). In the south-east and south-west, lobate moraines with splaying geometries, flanked by marginal meltwater channels (Figs. 7b, 8, and 9b), are common, resembling lobate land-terminating margins documented elsewhere on the soft-bedded (unconsolidated sediments) southern and eastern margins of the SIS (e.g. Kalm, 2012; Szuman et al., 2021) and Laurentide Ice Sheet (Margold et al., 2015). Ice margin signatures in the southern sector often comprise overprinted lobes interpreted as arising from ice margin oscillations and re-advances (Figs. 6 and 9b) along with switches in flow orientation and changing lobe positions during the overall retreat (see Pedersen, 2000; Kjær et al., 2003; Gehrmann and Hardig, 2018).
The presence of ice margin meltwater channels in Denmark, Germany, Poland, Kaliningrad, Lithuania, and the present-day coastal zone indicates that the marginal environment in this phase of retreat was terrestrial (Fig. 4) (see Uścinowicz, 1999). Flat-topped ice-contact fans in the southernmost sector (i.e. Darss Sill–Falster–Rügen Plain and Pomeranian Bay; Figs. 7d and 9b) indicate the positions of ice margins (Lemke et al., 1995, and references therein) and deposition of fine sediments in a shallow glaciolacustrine environment once the ice margin stepped back north of the present-day coast. While these ice-contact landforms resemble the shape of grounding zone wedges (see Batchelor and Dowdeswell, 2015), the water depth, only up to 30 m along the coast (Uścinowicz, 2003), is considered too shallow for ice shelf development. However, we note here the strong resemblance of the features we map to the Pas Moraine in Manitoba, which has recently been re-interpreted as a grounding zone wedge formed in the glacial Lake Agassiz (Gauthier et al., 2022).
In the southern part of the study area, numerous ice-dammed lakes have been reconstructed in and adjacent to the Baltic depression from sedimentological investigations (Houmark-Nielsen and Kjær, 2003; Larsen et al., 2009) and the identification of palaeo-shorelines (Uścinowicz, 1999). The largest of these lakes is the Baltic Ice Lake (Björck, 1995; Uścinowicz, 2006; Rosentau et al., 2017). During the retreat of the SIS through the Baltic depression, the ice margin may have terminated on land or been grounded in an ice-dammed lake, and we suggest that the varying geomorphologies noted above likely reflect these terminal environments. During the early stage of the Baltic Ice Lake, the areas south of the Słupsk Furrow, Odra, and Adler banks were elevated above the lake water (Uścinowicz, 1999). North of prominent central Baltic ice-contact landforms, east of Öland, the conditions were suitable for GZW (Fig. 7i) formation. Further north, parallel MSGLs are aligned within the deeper depressions (Fig. 9c). Here, specific MSGL sets cannot be conclusively linked to ice margin landforms. Given their highly parallel nature, alignment with the orientations of depressions that are up to 240 m deep, and overprinting by iceberg plough marks, we suggest that these landforms are indicative of a water-terminating ice margin with icebergs calving either directly at the ice front or from an ice shelf that might have existed over the deeper sections of the Baltic Ice Lake. The change from the plough-mark-free southern sector (Fig. 4) to numerous plough marks in the deeper (up to 220 m b.s.l.) northern sector likely reflects a transition from a land-terminating or shallow lacustrine margin to a deeper aqueous calving margin. Similar parallel corridors of MSGLs (i.e. not splaying) have been found at former marine-terminating margins of the Antarctic Ice Sheet (Livingstone et al., 2012). This is consistent with Noormets and Flodén (2002b), who suggested an ice thickness of 180 m at the grounding line, with a floating ice shelf over Fårö Deep (see Fig. 1 for the location). The distal slopes of elevated sediments at pinning points, especially along the klints, are covered with plough marks (Fig. 7f; see also Karpin et al., 2021), indicating either a long still-stand or that the calving processes were intensive, and the icebergs were smaller than the full depth of ice, allowing them to pass through topographic barriers.
5.3 What was the role of bedrock structures in controlling stepped retreat?
Glaciolacustrine sediments are widespread in the Baltic (Boulton and Jones, 1979; Boulton et al., 1985; Noormets and Flodén, 2002b, a; Uścinowicz, 1999). However, there are locations where bedrock is found to outcrop at the seafloor, including at the island of Bornholm and the klints in the northern Baltic (Fig. 1; Tuuling and Flodén, 2016). These outcrops are generally the result of more resistant geological units producing topographic protrusions. The topographic relief associated with the klints is associated with ice-contact landforms (Figs. 8 and 9d), including outwash fans (Noormets and Flodén, 2002b), GZWs and moraines (e.g. Fig. 7f), and tunnel valleys (Fig. 4). Lineations further north splay according to the klints' position (Fig. 9d) and can be incorporated into a stepped pattern of retreat. The quasi-regular pattern of ice margin positions (Fig. 8) gives an impression of stepped recession. In our interpretation, stepped retreat could be related to the presence of pinning points formed by the klints and other topographic or bathymetric features (see Boyce et al., 2017; Noormets and Flodén, 2002b). In the study area, the presence of ice-contact landforms along the higher-elevated Darss Sill–Falster Rügen Plain and Odra Bank (Figs. 7d, 9b), in the central Baltic (Fig. 7e; near the Devonian Klint from Fig. 1), along the klints (Figs. 7f and 9d, f; see also Noormets and Flodén, 2002b) north of Bornholm (Fig. 9e), is consistently associated with these geological structures (see Kramarska, 1998; Noormets and Flóden, 2002a; Jensen et al., 2017). The Darss Sill and Odra Bank have hard bedrock elevated by ca. 30 to 50 m compared to the Arkona and Bornholm basins (Jensen, 1995; Tomczak, 1995; Flodén et al., 1996). In East Gotland Basin the ice was steered and pinned by the higher-elevation Devonian Klint to the east (see Fig. 1, where the limits of East Gotland Basin follow the geology) and the extension of the Gotland ridge to the west. Thus, significant (the most prominent zones of sediment accumulation along the Baltic depression) accumulation of unconsolidated sediments along geological steps indicates the importance of pinning points in ice stabilisation (e.g. Favier et al., 2016; Still and Hulbe, 2021). The only prominent high on the Baltic bed where we do not map accumulations of glaciogenic sediments is Åland Sill, possibly because of patchier sediment availability over the crystalline bedrock.
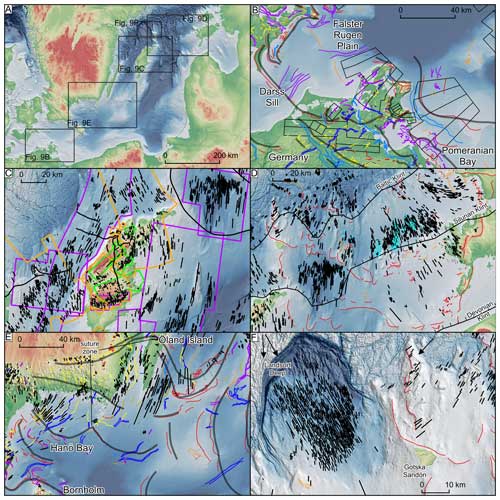
Figure 9(a) Locations of panels (b)–(f). The DEMs (a–f) are based on EU-DEM and EMODnet if not stated otherwise (see Table 2). (b) Lobate ice margin positions along the German coast, with a corresponding lobate pattern of flow sets and onshore–offshore ice-contact landforms (the legend is indicated in Fig. 4), and (c) highly parallel MSGLs in the bathymetric depressions (purple polygons). The parallel MSGLs indicate ice stream widths of roughly 30–60 km, with a maximum up to 95 km. Notice that splaying lineation patterns are rare in this location. The orientation of ice flow within the classified flow sets is indicated by colours: violet for N–S to NNE–SSW (possibly the oldest), orange for NW–SE (younger), and green for NE–SW to ENE–WSW (the youngest). The flow-set pattern underlines the interplay between ice margins with Swedish-oriented and Bothnian-oriented ice sources. The onshore DEM is provided by © Lantmäteriet (see Table 2). (d) Ice margins associated with topographic highs formed by the klints (black lines). Notice the highly lobate shape of ice-contact landforms that fits the positions of the klints. Glacial lineations have splaying patterns, especially down-ice from the Baltic Klint. The onshore DEM was provided by Estonian Land Board 2021 (see Table 2). (e) Suture zones in Hanö Bay, Öland, and the central Baltic. Note the ice splitting into two lobes, indicated by the pattern of eskers (yellow) and tunnel valleys (blue) and by the positions of ice-contact landforms (red) especially clearly visible to the east (a swarm of “red” margins; here the East and West Gotland lobes are in contact). The onshore DEM is provided by © Lantmäteriet (see Table 2). (f) Glacial lineations in the vicinity of Landsort Deep and Gotska Sandön indicate ice flow divergence towards the south-east and south from the Landsort Deep and south-west towards Gotska Sandön.
5.4 Was there an ice flow interaction between northern (Bothnian Basin) and north-western (Swedish) ice sources?
Past ice-sheet-scale investigations have inferred an ice lobe with a gently arcuate ice front spanning the width of the Baltic depression during northwards retreat, implying that ice was steered through the Gulf of Bothnia (Boulton et al., 2001). We confirm this Bothnian ice source from the north but also identify a Swedish ice source with a ∼ NW–SE orientation based on our ice margin and flow-set geometries (Figs. 6, 8, and 9c; see also Greenwood et al., 2024). These landforms record the interplay between two ice sources, with the high-resolution DEM from the islands of Gotland and northern Öland assisting in the identification of five independent ice flow directions (Figs. 6c and 9c). The zig-zag-like pattern of flow sets with ice flowing from NW–SE (Swedish source) and N–S to NE–SW (Bothnian source) indicates alternating activity of both ice sources. Our mapping indicates that the two ice lobes along West and East Gotland basins were in contact (Figs. 7e and 9e) in the earlier stages of deglaciation but with a later switch to dominance of the eastern lobe. The suture between the two lobes was located along Gotland (Fig. 8e) and extended towards Gotska Sandön in the north and ca. 75 km to the south. The multi-channelled meltwater system (Fig. 5i), widespread accumulation of glaciofluvial sediments (expressed here as ice-contact fans; see also Noormets and Flodén, 2002b, for evidence of glaciofluvial sediments along Gotska Sandön) and a concentration of MSGLs on both sides of Gotland are typical characteristics of suture zones (e.g. Punkari, 1997; Evans et al., 2018). We also identify more localised ice flow splitting north of the island of Gotland, indicated by diverging streamlined bedforms (N–S and more NW–SE) in Landsort Deep (Figs. 6a and 9f).
A Baltic-wide glacial landform-based map is presented, filling in a geographical gap in the record that has been speculated about by palaeo-glaciologists for over a century. The glacial landforms we map are interpreted as primarily recording phases of ice retreat through the Baltic rather than an extensive landform record related to the maximum ice extent phase. Instead of an often-interpreted or modelled accelerated ice flow zone spanning the Baltic depression (300 km), we provide landform evidence of narrower corridors of fast ice flow that we interpret as distinct and smaller ice streams (widths of 30 to 60 km, up to 95 km), consistent with later stages of deglaciation rather than the maximum stage. In the central Baltic, assemblages of landforms resemble lobate ice margins typical of terrestrial-style land systems, whereas locally, within the deeper bathymetric depressions, landform assemblages more typical of water-terminating ice margins are found. In these latter cases plough marks suggest significant ice evacuation by calving. Episodic rather than steady ice retreat is inferred based on ice margin positions associated with exposed bedrock structures that likely acted as pinning points. Our mapping identifies flow and ice margin geometries from both Swedish and northern Bothnian sources. We anticipate that our landform mapping and interpretations may be used as a framework for more detailed empirical studies by identifying targets to acquire high-resolution bathymetry and sediment cores and also for comparison with numerical ice sheet modelling.
The data set is available by request via e-mail at szuman@amu.edu.pl.
The supplement related to this article is available online at: https://doi.org/10.5194/tc-18-2407-2024-supplement.
IS and JZK conceived the project. IS led the project and wrote with CRD the initial version of the manuscript, which was subsequently improved by the contribution of all the co-authors. IS and JZK mapped the landforms. All the co-authors contributed to the interpretation of problematic areas.
The contact author has declared that none of the authors has any competing interests.
Publisher's note: Copernicus Publications remains neutral with regard to jurisdictional claims made in the text, published maps, institutional affiliations, or any other geographical representation in this paper. While Copernicus Publications makes every effort to include appropriate place names, the final responsibility lies with the authors.
This article is part of the special issue “Icy landscapes of the past”. It is not associated with a conference.
We are grateful to two anonymous reviewers and Sarah Greenwood for their valuable comments and Marek Ewertowski for constructive discussions.
This research has been supported by the Polish National Science Centre (NCN) (grant no. 2015/17/D/ST10/01975). Chris D. Clark and Christiaan R. Diemont were supported by the European Research Council, H2020 (PalGlac grant no. 787263).
This paper was edited by Irina Rogozhina and reviewed by two anonymous referees.
Ahlmann, H. W., Laurell, E., and Mannerfelt, C.: Det norrländska landskapet, Ymer, 62, 1–50, 1942.
All, T., Flodén, T., and Puura, V.: A complex model of Mesoproteozoic sedimentary and igneous suites in a graben setting north of Gotland, Baltic Sea, GFF, 128, 53–63, https://doi.org/10.1080/11035890601281053, 2006.
Amantov, A., Fjeldskaar, W., and Cathles, L.: Glacial Erosion/Sedimentation of the Baltic Region and the Effect on the Postglacial Uplift, in: The Baltic Sea Basin, edited by: Harff, J., Björck, S., and Hoth, P., Springer Berlin Heidelberg, Berlin, Heidelberg, 53–71, https://doi.org/10.1007/978-3-642-17220-5_3, 2011.
Batchelor, C. L. and Dowdeswell, J. A.: Ice-sheet grounding-zone wedges (GZWs) on high-latitude continental margins, Mar. Geol., 363, 65–92, https://doi.org/10.1016/j.margeo.2015.02.001, 2015.
Bennett, M. R.: Ice streams as the arteries of an ice sheet: their mechanics, stability and significance, Earth-Sci. Rev., 61, 309–339, https://doi.org/10.1016/S0012-8252(02)00130-7, 2003.
Björck, S.: A review of the history of the Baltic Sea, 13.0–8.0 ka BP, Quatern. Int., 27, 19–40, https://doi.org/10.1016/1040-6182(94)00057-C, 1995.
Björck, S., Dennegård, B., and Sandgren, P.: The marine stratigraphy of the Hanö Bay, SE Sweden, based on different sediment stratigraphic methods, Geol. Fören. Stock. För., 112, 265–280, https://doi.org/10.1080/11035899009454774, 1990.
Boulton, G. S. and Clark, C. D.: A highly mobile Laurentide ice sheet revealed by satellite images of glacial lineations, Nature, 346, 813–817, https://doi.org/10.1038/346813a0, 1990.
Boulton, G. S. and Jones, A. S.: Stability of Temperate Ice Caps and Ice Sheets Resting on Beds of Deformable Sediment, J. Glaciol., 24, 29–43, https://doi.org/10.3189/S0022143000014623, 1979.
Boulton, G. S., Smith, G. D., Jones, A. S., and Newsome, J.: Glacial geology and glaciology of the last mid-latitude ice sheets, J. Geol. Soc., 142, 447–474, https://doi.org/10.1144/gsjgs.142.3.0447, 1985.
Boulton, G. S., Dongelmans, P., Punkari, M., and Broadgate, M.: Palaeoglaciology of an ice sheet through a glacial cycle: the European ice sheet through the Weichselian, Quaternary Sci. Rev., 20, 591–625, https://doi.org/10.1016/S0277-3791(00)00160-8, 2001.
Boyce, E. S., Motyka, R. J., and Truffer, M.: Flotation and retreat of a lake-calving terminus, Mendenhall Glacier, southeast Alaska, USA, J. Glaciol., 53, 211–224, https://doi.org/10.3189/172756507782202928, 2017.
Catania, G., Hulbe, C., Conway, H., Scambos, T. A., and Raymond, C. F.: Variability in the mass flux of the Ross ice streams, West Antarctica, over the last millennium, J. Glaciol., 58, 741–752, 10.3189/2012JoG11J219, 2012.
Clark, C. D.: Remote Sensing Scales Related To The Frequency Of Natural Variation: An Example From Paleo-ice Flow In Canada, IEEE T. Geosci. Remote, 28, 503–508, https://doi.org/10.1109/TGRS.1990.572932, 1990.
Clark, P. U. and Walder, J. S.: Subglacial drainage, eskers, and deforming beds beneath the Laurentide and Eurasian ice sheets, GSA Bulletin, 106, 304–314, https://doi.org/10.1130/0016-7606(1994)106<0304:SDEADB>2.3.CO;2, 1994.
Cuzzone, J. K., Clark, P. U., Carlson, A. E., Ullman, D. J., Rinterknecht, V. R., Milne, G. A., Lunkka, J.-P., Wohlfarth, B., Marcott, S. A., and Caffee, M.: Final deglaciation of the Scandinavian Ice Sheet and implications for the Holocene global sea-level budget, Earth Planet. Sci. Lett., 448, 34–41, https://doi.org/10.1016/j.epsl.2016.05.019, 2016.
Dewald, N., Livingstone, S. J., and Clark, C. D.: Subglacial meltwater routes of the Fennoscandian Ice Sheet, J. Maps, 18, 382–396, https://doi.org/10.1080/17445647.2022.2071648, 2022.
Dorokhov, D. V., Dorokhova, E. V., and Sivkov, V. V.: Iceberg and ice-keel ploughmarks on the Gdansk-Gotland Sill (south-eastern Baltic Sea), Geo-Mar. Lett., 38, 83–94, https://doi.org/10.1007/s00367-017-0517-3, 2018.
Dowling, T. P. F., Spagnolo, M., and Möller, P.: Morphometry and core type of streamlined bedforms in southern Sweden from high resolution LiDAR, Geomorphology, 236, 54–63, https://doi.org/10.1016/j.geomorph.2015.02.018, 2015.
Eissmann, L.: Rhombenporphyrgeschiebe in Elster und Saalemoränen des Leipziger Raumes, Abh. Ber. Naturkund. Mus. Mauritianum Altenbg., 5, 37–46, 1967.
EMODnet Bathymetry Consortium: EMODnet Digital Bathymetry (DTM), European Marine Observation and Data Network Bathymetry Consortium, https://doi.org/10.12770/ff3aff8a-cff1-44a3-a2c8-1910bf109f85, 2022.
Enquist, F.: Die glaziale Entwicklungsgeschichte Nordwestskandinaviens, Sveriges Geologiska Undersökning Serie C 285, Kungl. boktryckeriet. P.A. Norstedt & Söner, Stockholm, 1918.
Evans, D. J. A., Dinnage, M., and Roberts, D. H.: Glacial geomorphology of Teesdale, northern Pennines, England: Implications for upland styles of ice stream operation and deglaciation in the British-Irish Ice Sheet, Proc. Geol. Assoc., 129, 697–735, https://doi.org/10.1016/j.pgeola.2018.05.001, 2018.
Favier, L., Pattyn, F., Berger, S., and Drews, R.: Dynamic influence of pinning points on marine ice-sheet stability: a numerical study in Dronning Maud Land, East Antarctica, The Cryosphere, 10, 2623–2635, https://doi.org/10.5194/tc-10-2623-2016, 2016.
Feldens, P., Diesing, M., Wilken, D., and Schwarzer, K.: Submarine eskers preserved on Adler Grund, south-western Baltic Sea, Baltica, 26, 137–144, https://doi.org/10.5200/baltica.2013.26.14 2013.
Flodén, T., Bjerkéus, M., Endler, R., and Lemke, W.: Cretaceous sedimentary bedrock of the Darss Sill area, southern Baltic Sea, GFF, 118, p. 80, https://doi.org/10.1080/11035899609546381, 1996.
Flodén, T., Bjerkéus, M., Sturkell, E., Gelumbauskaitė, Ž., Grigelis, A., Endler, R., and Lemke, W.: Distribution and seismic stratigraphy of glacially incised valleys in the southern part of the Baltic, Proceedings of the Fourth Marine Geological Conference–the Baltic, Sveriges Geologiska Undersökning, Ser. Ca, 86, 43–49, ISBN 9171585532, 1997.
Fowler, A. C. and Johnson, C.: Ice-sheet surging and ice-stream formation, Ann. Glaciol., 23, 68–73, https://doi.org/10.3189/S0260305500013276, 1996.
Frydrych, M.: Morphology of eskers in Poland, southward of the Last Glacial Maximum, Geomorphology, 415, 108418, https://doi.org/10.1016/j.geomorph.2022.108418, 2022.
Gandy, N., Gregoire, L. J., Ely, J. C., Cornford, S. L., Clark, C. D., and Hodgson, D. M.: Exploring the ingredients required to successfully model the placement, generation, and evolution of ice streams in the British-Irish Ice Sheet, Quaternary Sci. Rev., 223, 105915, https://doi.org/10.1016/j.quascirev.2019.105915, 2019.
Gauthier, M. S., Breckenridge, A., and Hodder, T. J.: Patterns of ice recession and ice stream activity for the MIS 2 Laurentide Ice Sheet in Manitoba, Canada, Boreas, 51, 274–298, https://doi.org/10.1111/bor.12571, 2022.
Gehrmann, A. and Harding, C.: Geomorphological Mapping and Spatial Analyses of an Upper Weichselian Glacitectonic Complex Based on LiDAR Data, Jasmund Peninsula (NE Rügen), Germany, https://doi.org/10.3390/geosciences8060208, 2018.
Glückert, G.: Map of glacial striation of the Scandinavian ice sheet during the last (Weichsel) glaciation in northern Europe, B. Geol. Soc. Finland, 46, 1–8, https://doi.org/10.17741/bgsf/46.1.001, 1974.
Greenwood, S. L. and Clark, C. D.: Reconstructing the last Irish Ice Sheet 2: a geomorphologically-driven model of ice sheet growth, retreat and dynamics, Quaternary Sci. Rev., 28, 3101–3123, https://doi.org/10.1016/j.quascirev.2009.09.014, 2009.
Greenwood, S. L., Clason, C. C., Mikko, H., Nyberg, J., Peterson, G., and Smith, C. A.: Integrated use of LiDAR and multibeam bathymetry reveals onset of ice streaming in the northern Bothnian Sea, GFF, 137, 284–292, https://doi.org/10.1080/11035897.2015.1055513, 2015.
Greenwood, S. L., Clason, C. C., Nyberg, J., Jakobsson, M., and Holmlund, P.: The Bothnian Sea ice stream: early Holocene retreat dynamics of the south-central Fennoscandian Ice Sheet, Boreas, 46, 346–362, https://doi.org/10.1111/bor.12217, 2016.
Greenwood, S. L., Avery, R. S., Gyllencreutz, R., Regnéll, C., and Tylmann, K.: Footprint of the Baltic Ice Stream: geomorphic evidence for shifting ice stream pathways, Boreas, 53, 4–26, https://doi.org/10.1111/bor.12634, 2024.
Gripp, K.: Der Ablauf der Würm-Vereisung in der Senkungszone am Südrand Skandinaviens, Meyniana, 33, 9–22, 1981.
Hall, A. and van Boeckel, M.: Origin of the Baltic Sea basin by Pleistocene glacial erosion, GFF, 142, 237–252, https://doi.org/10.1080/11035897.2020.1781246, 2020.
Hermanowski, P., Piotrowski, J. A., and Szuman, I.: An erosional origin for drumlins of NW Poland, Earth Surf. Proc. Land., 44, 2030–2050, https://doi.org/10.1002/esp.4630, 2019.
Hindmarsh, R. C. A.: Consistent generation of ice-streams via thermo-viscous instabilities modulated by membrane stresses, Geophys. Res. Lett., 36, L06502, https://doi.org/10.1029/2008GL036877, 2009.
Holmlund, P. and Fastook, J.: A time dependent glaciological model of the Weichselian Ice Sheet, Quatern. Int., 27, 53–58, https://doi.org/10.1016/1040-6182(94)00060-I, 1995.
Holmström, L.: Öfversikt af den glaciala afslipningen i Sydskandinavien, Geol. Fören. Stock. För., 26, 241–316, https://doi.org/10.1080/11035890409445487, 1904.
Houmark-Nielsen, M. and Kjær, K. H.: Southwest Scandinavia, 40–15 kyr BP: palaeogeography and environmental change, J. Quaternary Sci., 18, 769–786, https://doi.org/10.1002/jqs.802, 2003.
Jakobsson, M., O'Regan, M., Gyllencreutz, R., and Flodén, T.: Seafloor terraces and semi-circular depressions related to fluid discharge in Stockholm Archipelago, Baltic Sea, Geol. Soc. Lond. Mem., 46, 305–306, https://doi.org/10.1144/M46.162, 2016.
Jakobsson, M., Stranne, C., O'Regan, M., Greenwood, S. L., Gustafsson, B., Humborg, C., and Weidner, E.: Bathymetric properties of the Baltic Sea, Ocean Sci., 15, 905–924, https://doi.org/10.5194/os-15-905-2019, 2019.
Jakobsson, M., O'Regan, M., Mörth, C.-M., Stranne, C., Weidner, E., Hansson, J., Gyllencreutz, R., Humborg, C., Elfwing, T., Norkko, A., Norkko, J., Nilsson, B., and Sjöström, A.: Potential links between Baltic Sea submarine terraces and groundwater seeping, Earth Surf. Dynam., 8, 1–15, https://doi.org/10.5194/esurf-8-1-2020, 2020.
Jensen, J. B.: Late Weichselian deglaciation pattern in the southwestern Baltic: Evidence from glacial deposits off the island of Møn, Denmark, B. Geol. Soc. Denmark, 40, 314–331, 1993.
Jensen, J. B., Moros, M., Endler, R., and Members, I. E.: The Bornholm Basin, southern Scandinavia: a complex history from Late Cretaceous structural developments to recent sedimentation, Boreas, 46, 3–17, https://doi.org/10.1111/bor.12194, 2017.
Jögensen, F. and Piotrowski, J. A.: Signature of the Baltic Ice Stream on Funen Island, Denmark during the Weichselian glaciation, Boreas, 32, 242–255, https://doi.org/10.1111/j.1502-3885.2003.tb01440.x, 2003.
Jørgensen, F. and Sandersen, P. B. E.: Buried and open tunnel valleys in Denmark – erosion beneath multiple ice sheets, Quaternary Sci. Rev., 25, 1339-1363, https://doi.org/10.1016/j.quascirev.2005.11.006, 2006.
Kalm, V.: Ice-flow pattern and extent of the last Scandinavian Ice Sheet southeast of the Baltic Sea, Quaternary Sci. Rev., 44, 51–59, https://doi.org/10.1016/j.quascirev.2010.01.019, 2012.
Karpin, V., Heinsalu, A., and Virtasalo, J. J.: Late Pleistocene iceberg scouring in the north-eastern Baltic Sea, west of Estonia, Mar. Geol., 438, 106537, https://doi.org/10.1016/j.margeo.2021.106537, 2021.
Kehew, A. E., Piotrowski, J. A., and Jørgensen, F.: Tunnel valleys: Concepts and controversies – A review, Earth-Sci. Rev., 113, 33–58, https://doi.org/10.1016/j.earscirev.2012.02.002, 2012.
Kirkham, J. D., Hogan, K. A., Larter, R. D., Arnold, N. S., Ely, J. C., Clark, C. D., Self, E., Games, K., Huuse, M., Stewart, M. A., Ottesen, D., and Dowdeswell, J. A.: Tunnel valley formation beneath deglaciating mid-latitude ice sheets: Observations and modelling, Quaternary Sci. Rev., 323, 107680, https://doi.org/10.1016/j.quascirev.2022.107680, 2022.
Kjær, K. H., Houmark-Nielsen, M., and Richardt, N.: Ice-flow patterns and dispersal of erratics at the southwestern margin of the last Scandinavian Ice Sheet: signature of palaeo-ice streams, Boreas, 32, 130–148, https://doi.org/10.1111/j.1502-3885.2003.tb01434.x, 2003.
Kleman, J., Hättestrand, C., Borgström, I., and Stroeven, A.: Fennoscandian palaeoglaciology reconstructed using a glacial geological inversion model, J. Glaciol., 43, 283–299, https://doi.org/10.3189/S0022143000003233, 1997.
Kleman, J., Stroeven, A. P., and Lundqvist, J.: Patterns of Quaternary ice sheet erosion and deposition in Fennoscandia and a theoretical framework for explanation, Geomorphology, 97, 73–90, https://doi.org/10.1016/j.geomorph.2007.02.049, 2008.
Klingberg, F. and Larsson, O.: Maringeologiska undersökningar av erosionsrännor i Kalmarsund, Sveriges Geologiska Undersökning, Rapport 2017, 13, 2017.
Kramarska, R.: Origin and development of the Odra Bank in the light of the geologic structure and radiocarbon dating, Geol. Q., 42, 277–288, 1998.
Lampe, R., Naumann, M., Meyer, H., Janke, W., and Ziekur, R.: Holocene Evolution of the Southern Baltic Sea Coast and Interplay of Sea-Level Variation, Isostasy, Accommodation and Sediment Supply, in: The Baltic Sea Basin, edited by: Harff, J., Björck, S., and Hoth, P., Springer Berlin Heidelberg, Berlin, Heidelberg, 233–251, https://doi.org/10.1007/978-3-642-17220-5_12, 2011.
Larsen, N. K., Knudsen, K. L., Krohn, C. F., Kronborg, C., Murray, A. S., and Nielsen, O. L. E. B.: Late Quaternary ice sheet, lake and sea history of southwest Scandinavia – a synthesis, Boreas, 38, 732–761, https://doi.org/10.1111/j.1502-3885.2009.00101.x, 2009.
Larson, G. J., Lawson, D. E., Evenson, E. B., Alley, R. B., Knudsen, Ó., Lachniet, M. S., and Goetz, S. L.: Glaciohydraulic supercooling in former ice sheets?, Geomorphology, 75, 20–32, https://doi.org/10.1016/j.geomorph.2004.12.009, 2006.
Lemke, W. and Kuijpers, A.: Late Pleistocene and early Holocene paleogeography of the Darss Sill area, southwestern Baltic, Quatern. Int., 27, 73–81, https://doi.org/10.1016/1040-6182(94)00063-B, 1995.
Livingstone, S. J., Ó Cofaigh, C., Stokes, C. R., Hillenbrand, C.-D., Vieli, A., and Jamieson, S. S. R.: Antarctic palaeo-ice streams, Earth-Sci. Rev., 111, 90–128, https://doi.org/10.1016/j.earscirev.2011.10.003, 2012.
Ljunger, E.: Isdelarstudier vid polcirkeln, Geol. För. Stock. För., 65, 198–210, 1943.
Madsen, V.: Om indelingen af de danske kvartærdannelser, Fören, 5, 1–22, 1898.
Margold, M., Jansson, K. N., Kleman, J., Stroeven, A. P., and Clague, J. J.: Retreat pattern of the Cordilleran Ice Sheet in central British Columbia at the end of the last glaciation reconstructed from glacial meltwater landforms, Boreas, 42, 830–847, https://doi.org/10.1111/bor.12007, 2013.
Margold, M., Stokes, C. R., and Clark, C. D.: Ice streams in the Laurentide Ice Sheet: Identification, characteristics and comparison to modern ice sheets, Earth-Sci. Rev., 143, 117–146, https://doi.org/10.1016/j.earscirev.2015.01.011, 2015.
Noormets, R. and Flodén, T.: Glacial deposits and Late Weichselian ice-sheet dynamics in the northeastern Baltic Sea, Boreas, 31, 36–56, https://doi.org/10.1111/j.1502-3885.2002.tb01054.x, 2002a.
Noormets, R. and Flodén, T.: Glacial deposits and ice-sheet dynamics in the north-central Baltic Sea during the last deglaciation, Boreas, 31, 362–377, https://doi.org/10.1111/j.1502-3885.2002.tb01080.x, 2002b.
Obst, K., Nachtweide, C., and Müller, U.: Late Saalian and Weichselian glaciations in the German Baltic Sea documented by Pleistocene successions at the southeastern margin of the Arkona Basin, Boreas, 46, 18–33, https://doi.org/10.1111/bor.12212, 2017.
Patton, H., Hubbard, A., Andreassen, K., Winsborrow, M., and Stroeven, A. P.: The build-up, configuration, and dynamical sensitivity of the Eurasian ice-sheet complex to Late Weichselian climatic and oceanic forcing, Quaternary Sci. Rev., 153, 97–121, https://doi.org/10.1016/j.quascirev.2016.10.009, 2016.
Patton, H., Hubbard, A., Andreassen, K., Auriac, A., Whitehouse, P. L., Stroeven, A. P., Shackleton, C., Winsborrow, M., Heyman, J., and Hall, A. M.: Deglaciation of the Eurasian ice sheet complex, Quaternary Sci. Rev., 169, 148–172, https://doi.org/10.1016/j.quascirev.2017.05.019, 2017.
Payne, A. J.: Dynamics of the Siple Coast ice streams, west Antarctica: Results from a thermomechanical ice sheet model, Geophysical Research Letters, 25, 3173-3176, https://doi.org/10.1029/98GL52327, 1998.
Perini, L., Missiaen, T., Ori, G. G., and de Batist, M.: Seismic stratigraphy of Late Quaternary glacial to marine sediments offshore Bornholm, southern Baltic Sea, Sediment. Geol., 102, 3–21, https://doi.org/10.1016/0037-0738(95)00056-9, 1996.
Poprawa, P., Šliaupa, S., Stephenson, R., and Lazauskien, J.: Late Vendian–Early Palæozoic tectonic evolution of the Baltic Basin: regional tectonic implications from subsidence analysis, Tectonophysics, 314, 219–239, https://doi.org/10.1016/S0040-1951(99)00245-0, 1999.
Punkari, M.: Glacial and glaciofluvial deposits in the interlobate areas of the Scandinavian ice sheet, Quaternary Sci. Rev., 16, 741–753, https://doi.org/10.1016/S0277-3791(97)00020-6, 1997.
Ringberg, B.: Late Weichselian geology of southernmost Sweden, Boreas, 17, 243–263, https://doi.org/10.1111/j.1502-3885.1988.tb00554.x, 1988.
Rosentau, A., Bennike, O., Uścinowicz, S., and Miotk-Szpiganowicz, G.: The Baltic Sea Basin, in: Submerged Landscapes of the European Continental Shelf, edited by: Flemming, N. C., Harff, J., Moura, D., Burgess, A., and Bailey, G. N., 103–133, https://doi.org/10.1002/9781118927823.ch5, 2017.
Schäfer, W., Hübscher, C., and Sopher, D.: Seismic stratigraphy of the Klints Bank east of Gotland (Baltic Sea): a giant drumlin sealing thermogenic hydrocarbons, Geo-Mar. Lett., 41, 9, https://doi.org/10.1007/s00367-020-00683-3, 2021.
Smith, M. J. and Clark, C. D.: Methods for the visualization of digital elevation models for landform mapping, Earth Surf. Proc. Land., 30, 885–900, https://doi.org/10.1002/esp.1210, 2005.
Stephan, H.-J.: The Young Baltic advance in the western Baltic depression, Geol. Q., 45, 359–363, 2001.
Still, H. and Hulbe, C.: Mechanics and dynamics of pinning points on the Shirase Coast, West Antarctica, The Cryosphere, 15, 2647–2665, https://doi.org/10.5194/tc-15-2647-2021, 2021.
Stokes, C. R.: Geomorphology under ice streams: Moving from form to process, Earth Surf. Proc. Land., 43, 85–123, https://doi.org/10.1002/esp.4259, 2018.
Stroeven, A. P., Hättestrand, C., Kleman, J., Heyman, J., Fabel, D., Fredin, O., Goodfellow, B. W., Harbor, J. M., Jansen, J. D., Olsen, L., Caffee, M. W., Fink, D., Lundqvist, J., Rosqvist, G. C., Strömberg, B., and Jansson, K. N.: Deglaciation of Fennoscandia, Quaternary Sci. Rev., 147, 91–121, https://doi.org/10.1016/j.quascirev.2015.09.016, 2016.
Svendsen, J. I., Alexanderson, H., Astakhov, V. I., Demidov, I., Dowdeswell, J. A., Funder, S., Gataullin, V., Henriksen, M., Hjort, C., Houmark-Nielsen, M., Hubberten, H. W., Ingólfsson, Ó., Jakobsson, M., Kjær, K. H., Larsen, E., Lokrantz, H., Lunkka, J. P., Lyså, A., Mangerud, J., Matiouchkov, A., Murray, A., Möller, P., Niessen, F., Nikolskaya, O., Polyak, L., Saarnisto, M., Siegert, C., Siegert, M. J., Spielhagen, R. F., and Stein, R.: Late Quaternary ice sheet history of northern Eurasia, Quaternary Sci. Rev., 23, 1229–1271, https://doi.org/10.1016/j.quascirev.2003.12.008, 2004.
Sviridov, N. I. and Emelyanov, E. M.: Lithofacial complexes of quaternary deposits in the central and southeastern baltic sea, Lithol. Miner. Resour., 35, 211–231, https://doi.org/10.1007/BF02821956, 2000.
Szuman, I., Kalita, J. Z., Ewertowski, M. W., Clark, C. D., and Livingstone, S. J.: Dynamics of the last Scandinavian Ice Sheet's southernmost sector revealed by the pattern of ice streams, Boreas, 50, 764–780, https://doi.org/10.1111/bor.12512, 2021.
Tomczak, A.: Geological Structure and Holocene Evolution of the Polish Coastal Zone, J. Coast. Res., 22, 15–31, 1995.
Tuuling, I. and Flodén, T.: The structure and relief of the bedrock sequence in the Gotland-Hiiumaa area, northern Baltic Sea, GFF, 123, 35–49, https://doi.org/10.1080/11035890101231035, 2001.
Tuuling, I. and Flodén, T. O. M.: Silurian reefs off Saaremaa and their extension towards Gotland, central Baltic Sea, Geolog. Mag., 150, 923–936, https://doi.org/10.1017/S0016756813000101, 2013.
Tuuling, I. and Flodén, T.: The Baltic Klint beneath the central Baltic Sea and its comparison with the North Estonian Klint, Geomorphology, 263, 1–18, https://doi.org/10.1016/j.geomorph.2016.03.030, 2016.
Tylmann, K. and Uścinowicz, S.: Timing of the last deglaciation phases in the southern Baltic area inferred from Bayesian age modeling, Quaternary Sci. Rev., 287, 107563, https://doi.org/10.1016/j.quascirev.2022.107563, 2022.
Uścinowicz, S.: Southern Baltic area during the last deglaciation Geol. Q., 43, 137–148, 1999.
Uścinowicz, S.: Relative sea level changes, glacio-isostatic rebound and shoreline displacement in the Southern Baltic, Polish Geological Institute Special Papers, 10, 1–79, 2003.
Uścinowicz, S.: A relative sea-level curve for the Polish Southern Baltic Sea, Quatern. Int., 145–146, 86–105, https://doi.org/10.1016/j.quaint.2005.07.007, 2006.
Woldstedt, P. and Duphorn, K.: Norddeutschland und angrenzende Gebiete im Eiszeitalter, Stuttgart, ISBN 3874251225, 1974.
Woźniak, P. P. and Czubla, P.: The Late Weichselian glacial record in northern Poland: A new look at debris transport routes by the Fennoscandian Ice Sheet, Quatern. Int., 386, 3–17, https://doi.org/10.1016/j.quaint.2015.01.014, 2015.
Zeise, O.: Beitrag zur Kenntnis der Ausbreitung, sowie besonders der Bewegungsrichtung des nordeuropäischen Inlandeises in diluvialer Zeit, Inaugural-Dissertation, Albertus-Universität, Königsberg, Preußen, 1889.
- Abstract
- Introduction
- Background: the role of the Baltic depression during the Last Glacial
- Methods
- Results – geomorphology and landforms of the Baltic Sea area
- Discussion
- Conclusions
- Data availability
- Author contributions
- Competing interests
- Disclaimer
- Special issue statement
- Acknowledgements
- Financial support
- Review statement
- References
- Supplement
- Abstract
- Introduction
- Background: the role of the Baltic depression during the Last Glacial
- Methods
- Results – geomorphology and landforms of the Baltic Sea area
- Discussion
- Conclusions
- Data availability
- Author contributions
- Competing interests
- Disclaimer
- Special issue statement
- Acknowledgements
- Financial support
- Review statement
- References
- Supplement