the Creative Commons Attribution 4.0 License.
the Creative Commons Attribution 4.0 License.
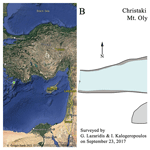
Brief communication: Tritium concentration and age of firn accumulation in an ice cave of Mount Olympus (Greece)
Georgios Lazaridis
Konstantinos Stamoulis
Despoina Dora
Iraklis Kalogeropoulos
Konstantinos P. Trimmis
Firn from an ice cave in the highest mountain of Greece, Mount Olympus, was sampled and analyzed to determine the tritium content in order to estimate rates of accumulation and to date the ice plug. The presence of a sharp raise of tritium content indicating the nuclear testing era was expected to be preserved into ice beds. Tritium concentrations were found to vary from 0.9 to 11 TU. This peak did not appear in the analyzed samples, providing an upper age limit of less than 50 years for the oldest sampled layer. It is suggested that the rate of melting is responsible for the absence of older firn layers.
- Article
(2491 KB) - Full-text XML
-
Supplement
(1048 KB) - BibTeX
- EndNote
Ice caves or caves hosting perennial ice accumulations (Perşoiu and Lauritzen, 2018) in Greece are scattered throughout the country's latitude. In total, 76 records of ice caves in Greece have been processed according to the Luetscher and Jeannin (2004) classification scheme and were examined according to climatological criteria, particularly the prevailing air dynamics and glaciological characteristics, such as the type of ice, by Lazaridis et al. (2018). On Mount Olympus, which is the highest mountain of the country, all the ice caves are classified as “static with firn”, where “static” is interpreted as single-entrance caves that form single down-sloping conduits. Ice of these caves, particularly those on the eastern slopes of Olympus, has been exploited for years between the end of the 19th century and the 1950s to provide ice to villages and towns in the foothills of the mountain and across the southwestern Greek Macedonia.
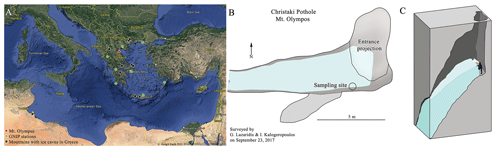
Figure 1(a) Location of Mt. Olympus and the sampled cave Christaki Pothole; tritium monitoring stations (IAEA/WMO, 2022, and the GNIP (Global Network of Isotopes in Precipitation) database, accessible at https://nucleus.iaea.org/wiser, last access: 21 December 2022) and other mountains of Greece with ice caves (satellite image source from © Google Earth 2022). (b) Ground plan with the sampling site depicted and (c) 3D representation of the cave.
In order to determine the age of the firn accumulation in these caves, measurements of the concentration of tritium in the ice were carried out at the Christaki Pothole in Mount Olympus. Tritium is a hydrogen isotope that decays, emitting beta particles of very low energy ( 18.6 keV) with a half-life of 4500±8 d (Lucas and Unterweger, 2000; Ehhalt et al., 2002). Tritium is produced in the upper atmosphere by the interaction of cosmic rays with the atoms of the atmosphere. This mechanism introduces tritium into the water cycle by the form of tritiated water and the normal concentration of tritium in precipitation is found to be 5–10 TU (tritium units, 1 TU = 0.11919 Bq L−1, Terzer-Wassmuth et al., 2022). During the nuclear era of the late 1950s and early 1960s, hydrogen bomb detonations introduced huge amounts of tritium into the atmosphere, resulting in a sharp peak of tritium concentration in precipitation all over the world (Martell, 1963), especially in the Northern Hemisphere where tritium concentrations were increased up to 6000 TU which was reached in 1963 (Cauquoin et al., 2016). In Greece, a maximum of 3550 TU was also observed in 1963. The tritium peaks in precipitation of the early 1960s are expected to be preserved in ice sections, if they have not melted since then. Previous studies have dated or provided constraints on the age of the ice deposits by using the presence (Kern et al., 2009; Borsato et al., 2004; Kern et al., 2018) or absence (Kern et al., 2011) of the tritium peak.
The cave under investigation (Fig. 1; and Supplement) is located on the northwestern slope of Mount Olympus (40.06898∘ N, 22.31350∘ E) at an altitude of 2350 m, very close to the Christaki refuge. The sampling cave was first investigated in 2016 and was included in the cadastre of ice caves in Greece, under the name Christaki Pothole (Lazaridis et al., 2018). The cave was surveyed once every year, for 3 consecutive years (from 2016 to 2018) during summer or early autumn, and ice accumulation was detected in each survey. The entrance of the pit is about 10 m and continues with the floor inclining to the west. Most of the cave is covered by an ice plug that overlies limestone gravels and small blocks. The ice plug consists of accumulated firn and snow. When surveyed, it was 1.5 m thick below the entrance and progressively thickening westwards, reaching a maximal thickness of 4.5 m. The ice plug prevents any access to the westward continuation of the cave. The cave is hosted in the Cretaceous crystalline limestones with dolomite interferences (Latsoudas and Sonis, 1985). The limestone sequence has a total thickness of about 2650 m and consists of limestones that gradually transition to dolomite. While the eastern slopes of Olympus are affected by the maritime air masses, the western slopes of Mount Olympus act as a barrier to the hot and humid westerly air masses and thus experience enhanced orographic precipitation. Ice caves in Greece are directly influenced by the prevailing climatic conditions (Perşoiu et al., 2021). The amount of precipitation in combination with the high altitude leads to snowfalls even during the summer season (Sahsamanoglou, 1989). Snow accumulation has been measured to reach over 2.5 m of depth within wide topographic depressions of Olympus in altitudes higher than 2000 m (Styllas et al., 2016).
On 23 September 2017, 41 ice samples were collected from the Christaki Pothole. Each sample was collected from a 2 m high section (Fig. 2) with a portable cup drill of 5 cm diameter and about 2–3 cm depth, resulting in samples of 30–40 cm3 at 5 cm intervals and spanning from the top of the ice section to the cave floor. Samples with odd numbering were selected for tritium content determination. Measurements were performed at the Archaeometry Center of the University of Ioannina.
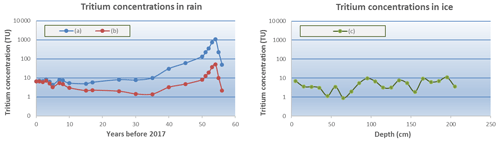
Figure 3Tritium concentration: the blue line (a) shows the annual mean values of tritium in rain samples from various monitoring stations in Greece (GNIP, IAEA, see Fig. 1 for the sites of the stations and Table S3 for the coordinates and altitude). The red line (b) shows the annual mean tritium values of line (a) corrected for decay at the year of ice sampling (2017). The green dots (c) show the tritium content in ice samples of odd numbering with depth, from the Christaki pothole.
Tritium measurements were conducted in a liquid scintillation analyzer (TR/SL 3700 Tricarb, Perkin Elmer). For each sample, 8 mL of melted ice, without electrolytic enrichment, was added to a low-potassium borosilicate glass vial, of 20 mL capacity, together with 12 mL of Ultima Gold LLT scintillation cocktail. The vial was closed and shaken to homogenize the solution and was typically measured for 1400 min. The background measurement was also recorded at the same batch of samples. In order to establish the detection limit of the method, several background measurements were pooled and a mean value of 1.200±0.006 cpm were selected as the representative value. The detection limit was calculated using the equation (TU), where σB= 0.006 cpm is the uncertainty for the background, eff = 0.26 the efficiency of the detector, V= 0.008 L the volume of the sample, 60 denotes minutes to seconds and 0.119919 Bq L−1 = 1 TU. The calculated value was DL = 1.2 TU.
The lack of well-distinguished layers precluded the estimation of the age of the samples extracted from the ice deposit by layer counting. However, during the sample processing, it was revealed that almost every ice sample contained remains of dust and soil which is the result of the surface debris deposited during the yearly cycle of snow accumulation during winter and partial melting during summer. Since the cave firn deposit was thought to have been accumulated for many decades, it was hypothesized that the abovementioned atmospheric tritium peak would be found in the melted ice samples. Instead, tritium concentrations were found to vary only from 0.9 to 11 TU. This range of tritium concentrations could be attributed to different initial tritium concentrations in snowfall, either a few years before the sampling year or even older. In comparison with tritium concentrations for selected years before the sampling year (2017), most of the tritium concentrations, corrected for decay, could result from precipitation up to 50 years before the sampling year, which corresponds to the calendar year 1967, when the mean annual tritium content was in the range of 130–230 TU (Fig. 3, Table S2).
Another consideration could be the case in which the deposited firn would be the result of the last decade of precipitation, when the annual variation of tritium concentration was in the same range (5–10 TU). In that case, the local maximums, observed in the tritium concentration through the samples, could be attributed to annual season maximums usually observed during the spring to summer months. This consideration could lead to 7–10 annual cycles observed in the preserved 2 m high ice bed, concluding an annual accumulation rate to the firn of about 20–30 cm yr−1. Finally, the 3H activity levels, found in the ice samples, could not be attributed to precipitation that fell before the 1950s, because in that case, higher concentrations remaining from the high tritium concentrations during the early 1960s should have been preserved into some of the measured samples. This gives an upper limit of the possible age of the ice layers that were sampled during the campaign of September 2017. Considering this upper limit of 50 years for the base of ice deposit, the corresponding mean winter ice layer thickness is at least 4 cm yr−1; the result is in accordance with the presence of debris in almost each sample as well as with findings in other ice caves. In the Monlesi ice cave, Switzerland, an annual accumulation rate 7–11 cm yr−1 was estimated based on tritium and 210Pb measurements (Luetscher et al., 2007) and in Ledena Pit, a Croatian ice cave, an average accumulation rate of up to 12 cm yr−1 during the period of 1963–1995 was also estimated (Kern et al., 2008). In other cases (Racine et al., 2022), the radiocarbon dating of organic remains in the firn reveal ages that span from modern times to almost 2700 BCE, depending on the morphology, the microenvironment and the height of the accumulated firn. For heights much larger than 2 m of the case presented here, varying from 5 to 27 m above the base of the firn, the estimated mean annual accumulation rates were much lower, varying from a few centimeters to less than 1 cm yr−1. However, the estimates of the present work do not date the onset of cave glaciation, nor times at which the cave may have been completely ice free. The ice plug in ice caves melts when it is in contact with the bedrock (Bella and Zelinka, 2018; Telbisz, 2019; Racine et al., 2022). Thus, the results are rather indicative of a melting rate that cannot support the preservation of ice that is older than 50 years. However, in deeper inaccessible parts of the cave, older ice may be preserved.
The Christaki Pothole provided a 2 m thick succession of ice samples that display relatively low concentration of tritium, without any pattern of an abrupt increase that could correspond to the tritium peak due to hydrogen bomb detonations in the early 1960s. This indicates that accumulated firn beds were younger than 50 years in 2017. The absence of earlier beds is suggested to be the result of the melting rate at the bottom of the ice plug, or the tritium peak may occur in ice beds that are not accessible due to the morphology of the Christaki Pothole.
All raw data are provided in the Supplement.
The supplement related to this article is available online at: https://doi.org/10.5194/tc-17-883-2023-supplement.
GL initiated the research; GL and KS organized fieldwork and prepared the manuscript with inputs from all authors; KS analyzed samples; GL and IK surveyed the cave and sampled the ice; GL, KS and IK did the field work; DD and KPT collected information.
The contact author has declared that none of the authors has any competing interests.
Publisher’s note: Copernicus Publications remains neutral with regard to jurisdictional claims in published maps and institutional affiliations.
We are deeply grateful to Sakis Ntavlis and Vasilis Sidiropoulos, cavers of the Hellenic Speleological Society, Department of Northern Greece for their contribution during the field work and sampling. Tanguy Racine and one anonymous referee are thanked for their comments and suggestions, as reviewers, which improved the paper.
This paper was edited by Regula Frauenfelder and reviewed by Tanguy Racine and one anonymous referee.
Bella, P. and Zelinka, J.: Ice caves in Slovakia, in: Ice caves, edited by: Persoiu, A. and Lauritzen, S.-E., Elsevier, 657–689, https://doi.org/10.1016/B978-0-12-811739-2.00029-2, 2018.
Cauquoin, A., Jean-Baptiste, P., Risi, C., Fourré, É., and Landais, A.: Modeling the global bomb tritium transient signal with the AGCM LMDZ-iso: A method to evaluate aspects of the hydrological cycle, J. Geophys. Res.-Atmos., 121, 12612–612629, 2016.
Borsato, A., Miorandi, R., and Flora, O.: I depositi di ghiaccio ipogei della Grotta dello Specchio e del Castelletto di Mezzo (Dolomiti di Brenta, Trentino): morfologia, età ed evoluzione recente, Studi Trent. Sci. Nat., Acta Geol., 81, 53–74, 2004.
Ehhalt, D. H., Rohrer, F., Schauffler, S., and Pollock, W., Tritiated water vapor in the stratosphere: Vertical profiles and residence time, J. Geophys. Res., 107, 4757, https://doi.org/10.1029/2001JD001343, 2002.
IAEA/WMO: GNIP (Global Network of Isotopes in Precipitation) database, https://nucleus.iaea.org/wiser, last access: 21 December 2022.
Kern, Z., Bočić, N., Horvatinčić, N., Fórizs, I., Nagy, B., and László, P.: Palaeoenvironmental records from ice caves of Velebit Mountains-Ledena Pit and Vukušić Ice Cave, Croatia, in: 3rd International Workshop on Ice Caves Proceedings, Kungur, 108–113, https://www.researchgate.net/publication/228488524_Palaeoenvironmental_records_from_ice_caves_of_Velebit_mountains-Ledena_pit_and_Vukusic_ice_cave_Croatia (last access: 13 February 2023), 2008.
Kern, Z., Molnár, M., Svingor, É., Perşoiu, A., and Nagy, B.: High-resolution, well-preserved tritium record in the ice of Bortig Ice Cave, Bihor Mountains, Romania, Holocene, 19, 729–736, 2009.
Kern, Z., Fórizs, I., Pavuza, R., Molnár, M., and Nagy, B.: Isotope hydrological studies of the perennial ice deposit of Saarhalle, Mammuthöhle, Dachstein Mts, Austria, The Cryosphere, 5, 291–298, https://doi.org/10.5194/tc-5-291-2011, 2011.
Kern, Z., Palcsu, L., Pavuza, R., and Molnár, M.: Age Estimates on the Deposition of the Cave Ice Block in the Saarhalle Dachstein-Mammoth Cave (Mammuthöhle, Austria) based on 3H and 14C, Radiocarbon, 60, 1379–1389, https://doi.org/10.1017/RDC.2018.96, 2018.
Latsoudas, C. and Sonis, C.: Kantariotissa-Litochoro sheet, Greece, Institute of Geology and Mineral Exploration, Greece, Geological map of Greece, scale , Athens, https://shop.geospatial.com/product/2NTPQ244G8064SY76Z GHB9TM23/88-Kontariotissa-Litochoro-Sheet-Greece-1-to-50000-Scale-Geological-Maps (last access: 13 February 2023), 1985.
Lazaridis, G., Theodosiadis, T., and Athanassopoulos, V.: Ice caves in Greece, Cave and Karst Science, 45, 33–38, 2018.
Lucas, L. L. and Unterweger, M. P.: Comprehensive Review and Critical Evaluation of the Half-Life of Tritium, J. Res. Natl. Inst. Stan., 105, 541–549, https://doi.org/10.6028/jres.105.043, 2000.
Luetscher, M. and Jeannin, P.-Y.: A process-based classification of alpine ice caves, Theoretical and Applied Karstology, 17, 5–10, 2004.
Luetscher, M., Bolius, D., Schwikowski, M., Schotterer, U., and Smart, P. L.: Comparison of techniques for dating of subsurface ice from Monlesi ice cave, Switzerland, J. Glaciol., 53, 374–384, 2007.
Martell, E. A.: On the inventory of artificial tritium and its occurrence in atmospheric methane, J. Geophys. Res., 68, 3759–3770, 1963.
Perşoiu, A. and Lauritzen, S.-E.: Ice caves, Elsevier, https://doi.org/10.1016/C2016-0-01961-7, 2018.
Perşoiu, A., Buzjak, N., Onaca, A., Pennos, C., Sotiriadis, Y., Ionita, M., Zachariadis, S., Styllas, M., Kosutnik, J., Hegyi, A., and Butorac, V.: Record summer rains in 2019 led to massive loss of surface and cave ice in SE Europe, The Cryosphere, 15, 2383–2399, https://doi.org/10.5194/tc-15-2383-2021, 2021.
Racine, T. M. F., Reimer, P. J., and Spötl, C. Multi-centennial mass balance of perennial ice deposits in Alpine caves mirrors the evolution of glaciers during the Late Holocene, Sci. Rep., 12, 11374, https://doi.org/10.1038/s41598-022-15516-9, 2022.
Sahsamanoglou, H. S.: Summer snowfalls over the mount Olympus area, Int. J. Climatol., 9, 309–319, 1989.
Styllas, M. N., Schimmelpfennig, I., Ghilardi, M., and Benedetti, L.: Geomorphologic and paleoclimatic evidence of Holocene glaciation on Mount Olympus, Greece, Holocene, 26, 709–721, 2016.
Telbisz, T.: Characteristics and Genesis of Subsurface Features in Glaciokarst Terrains, in: Glaciokarsts, Springer Geography, Springer, Cham, https://doi.org/10.1007/978-3-319-97292-3_5, 2019.
Terzer-Wassmuth, S., Araguás-Araguás, L. J., Copia, L., and Wassenaar, L. I.: High spatial resolution prediction of tritium (3H) in contemporary global precipitation, Sci. Rep., 12, 10271, https://doi.org/10.1038/s41598-022-14227-5, 2022.