the Creative Commons Attribution 4.0 License.
the Creative Commons Attribution 4.0 License.
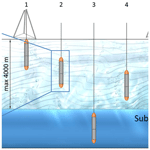
Brief communication: New sonde to unravel the mystery of polar subglacial lakes
Youhong Sun
Bing Li
Xiaopeng Fan
Yuansheng Li
Guopin Li
Haibin Yu
Hongzhi Li
Dongliang Wang
Nan Zhang
Da Gong
Rusheng Wang
Yazhou Li
The newly developed RECoverable Autonomous Sonde (RECAS) allows sampling and analysis of subglacial water while the subglacial lake remains isolated from the surface. The sonde was successfully tested in East Antarctica during the 2021–2022 field season: it reached the ice-sheet base at a depth of 200.3 m, sampled basal meltwater and measured its pressure, temperature, pH and conductivity and returned to the ice surface. The average downward penetration rate was 1.85 m h−1, and the average upward penetration rate was 2.94 m h−1. The successful test of a self-melting, recoverable probe in Antarctica marks further progress towards the clean exploration of subglacial water bodies.
- Article
(4152 KB) - Full-text XML
- BibTeX
- EndNote
The base of Antarctic and Greenland ice sheets, polar ice caps and valley glaciers demonstrates alternating warm-based and cold-based areas. According to a hybrid ice-sheet–ice-stream model, approximately 20 % of the Antarctic ice sheet is likely frozen to the bed, whereas the remainder is at the pressure-melting point (Pattyn, 2010). Subglacial meltwater will eventually collect in topographic hollows and trenches, forming a complicated subglacial hydrological system. The presence of a vast network of lakes, rivers and streams is verified by remote-sensing surveys that identify accumulations of liquid water. The latest global inventory has identified 773 subglacial lakes in total, which includes 675 from Antarctica, 64 from Greenland, 2 beneath the Devon Ice Cap, 6 beneath Iceland's ice caps and 26 from valley glaciers (Livingstone et al., 2022). The inventory is likely to increase as the surveys improve spatial coverage. The hydraulic potential reveals that some of the lakes could be hydraulically connected with each other and with the ocean, whereas the others remain isolated. Sealed from the Earth's atmosphere for many years, the subglacial aquatic environment, especially hydraulically sealed systems, may provide unique information about the microbial evolution, the past climate of the Earth and the formation of the ice sheets and glaciers (Pearce, 2009; Siegert et al., 2016). The discovery of subglacial aquatic environments has opened an entirely new area of science in a short period of time.
Although modern observations widely employ remote-sensing instruments to provide indirect indications of subglacial environment phenomena, the direct observation and sampling by drilling are still much needed for hydrological, chemical and microbiological studies. Drilling down and retrieving clean samples from deep subglacial lakes would provide scientists with a treasure trove comparable to the lunar rocks, which gave insight into the origins and evolution of our planet. Currently, four attempts were made to access, measure in situ properties and directly sample subglacial Antarctic lake environments.
In February 2012, a Russian team first penetrated subglacial Lake Vostok, the largest subglacial lake in Antarctica, at a depth of 3769.3 m. Operations allowed the lake water to enter and freeze within the lower part of the ice-core borehole, from which further coring recovered a frozen sample of surface lake water (Lukin and Vasiliev, 2014). Unfortunately, when subglacial water entered the borehole, it was contaminated by toxic drilling fluid (Alekhina et al., 2017). Second, a UK team tried to deploy a hot-water drill and sample the water column and sediments of subglacial Lake Ellsworth approximately 3000 m beneath the surface of the West Antarctic ice sheet (Siegert et al., 2014). Attempts to access this subglacial lake were unsuccessful in December 2012. The main reason for the failure relates to a subsurface cavity of water 300 m beneath the ice surface that could not be connected to the main drill hole. The circulation failure consequently resulted in insufficient water being available to continue drilling deeper. Third, in early 2013, and fourth, during the season of 2018–2019, US teams successfully drilled into subglacial lakes Whillans and Mercer, hydraulically active lakes at the coastal margin of West Antarctica, with hot-water circulation (Priscu et al., 2021; Tulaczyk et al., 2014). During penetration and sampling, the subglacial lakes were connected to the surface via a borehole. The ice thickness above these lakes is relatively shallow (800–1067 m), leaving the problem of clean sampling of the deep subglacial lakes still unsolved.
In 2019, a joint UK–Chile collaborative partnership was started for the exploration of subglacial Lake Centro de Estudios Científicos (CECs) at a depth of near 2650 m, also using a deep hot-water drilling system (Makinson et al., 2021). Plans included comprehensive field testing during the 2021–2022 austral summer at a site near the targeted subglacial lake. Unfortunately, because of the COVID19 pandemic, the CECs project has been terminated (Keith Makinson, personal communication, 2021). In addition, there have been several proposals for sampling subglacial lakes that did not go further than preliminary conceptual level (Blake and Price, 2002; Fleckenstein and Eustes, 2007).
In this study, we undertake field testing of a new instrument to sample subglacial lakes. We propose a freezing-in hot-point thermal drill (so-called Philberth probe) that is able to move towards the glacier base by melting ice while the melted water refreezes behind the probe. This type of probe was proposed by Philberth (1976) to study the temperature distribution in ice sheets. The most notable characteristic of the probe is that the wires used for the electrical power transmission to them and the signals transmission from them pay out of the advancing drill and became fixed in the refreezing meltwater above it, hence the probe only traveled one way. In the summer of 1968, the Philberth probe reached the remarkable depth of 1005 m at the Jarl-Joset station in Greenland. In the following years, nine similar freezing-in thermal probes were designed in different conceptual and testing phases, but all of them traveled one way (probes are reviewed in Talalay, 2020). In this paper, we present design and field tests of newly developed Philberth technology that travels back to the surface to sample subglacial lakes while the subglacial lake remains isolated from the surface.
Our proposed freezing-in probe – RECoverable Autonomous Sonde (RECAS) – is equipped with two electrically powered melting tips located at the upper and lower ends of the sonde (Talalay et al., 2014). Top and bottom thermal tips are totally identical, except for the central hole for the cable in the top thermal tip (Li et al., 2020). Thus, it can drill downwards and upwards and move in the borehole using an inner cable-recoiling mechanism similar to how a spider climbs on its silk line (Fig. 1). The sonde intends to explore subglacial aquatic environments with minimal chemical and microbial contamination.
Initially, the RECAS, power and communication systems are installed in a dedicated area of the ice surface above a subglacial lake. Prior to deployment, all downhole components are sterilized using a combination of chemical washing, hydrogen peroxide vapor and ultraviolet sterilization in China. Then, RECAS parts are assembled into independent modules in a clean room and packed into vacuum bags for shipment. The sterilization procedure follows the microbial control methods designed by the Lake Ellsworth Consortium for the UK subglacial sampling probe (Siegert et al., 2012), although the deployment procedure does not include assessment to provide data for control or verification of the cleanliness in the field.
Upon activating the lower melting tip, the sonde starts to melt down into the ice bed. Similar to the Philberth probe, the melted water is not recovered from the hole and refreezes behind the sonde. The power-signal cable is released from the coil inside the sonde. Motors and electronics are integrated in the pressure chamber while all other parts of the sonde are flooded. The coil motor slows down the paying out of the cable in such a way that the support point is above the center of gravity of the RECAS. Because of this, the sonde is inherently stable, and its attitude is always plumb.
The sonde rises and lowers using a dual-wheel capstan-driving mechanism. Three different motors are used to drive a capstan, drum with cable and level winder. The rotation of the capstan motor and corresponding moving speed of the sonde is automatically controlled depending on the signal from the load sensor. The rotation of the drum's motor is also automatically controlled according to the signal from the tension sensor installed between capstan and drum, in order to keep the cable tension in the predetermined range.
Sampling of relatively shallow subglacial lakes (500–1000 m) can be conducted during the summer season (1–2 months) and the system is supervised by personnel. However, the exploration of a deeply buried subglacial lake likely requires 4–6 months. In this case, it was proposed that the research personnel leave the site after deployment and the sonde operates as a fully autonomous system. The smart drilling system measures, controls and adapts to conditions throughout the drilling operation. An automatically controlled diesel generator provides power till the mission would be completed for at least 6 months in an unmanned mode. Coded data from the RECAS are transmitted to a computer on the surface and automatically sent via satellite communication to China for inspection. The connection with RECAS is established with Secure Shell (SSH) protocol, which is commonly used for secure remote login over an insecure network. The data include the main parameters of RECAS and the uninterrupted power supply system, which are packed and sent to the monitoring center in China at regular intervals (<1 min). Remote personnel can not only monitor the drilling process in the field but also send commands to intervene in the process at any time.
When the sonde enters the subglacial lake, it samples the water and examines water parameters such as pressure, temperature, pH and conductivity (Table 1). The pressure and temperature sensors are custom-made on the base of standard sensing elements, while we completely developed the conductivity sensor ourselves. The pH sensor is a standard sensor of AMT-6000 type consisting of glass and reference electrodes. Upon completing the sampling and monitoring, the coil motor is activated, and the top thermal tip is powered. The recovery of the sonde to the surface begins with spooling of the cable. Finally, the sonde reaches the surface and is ready for service to move to the next site. Therefore, once the sonde reaches the ice base, the subglacial lake remains isolated from the surface, reducing the risk of lake contamination.
One of the key steps of the project implementation was to find the optimal relationship between the sonde length, diameter and power consumption with the diameter and length (volume) of the used cable. Balance between the space for the 6 mm diameter cable and power requirements were found to be optimal when 900–1000 m of the cable is coiled onto the inner winch of the sonde. Finally, the RECAS working prototype (outer diameter 180 mm, length 7.275 m, weight 330 kg, maximum power consumption of heaters 8.82 kW) with a 500 m long cable (five winding layers) inside was built and prepared for laboratory and field tests (Fig. 2).
After numerous laboratory tests with melting tips (Li et al., 2020), cables (Zhang et al., 2021), electronic control systems (Peng et al., 2021), heating control systems (Yu et al., 2021) and tension sensors (Shi et al., 2021), the RECAS working prototype was finally prepared for Antarctic field tests during the 2021–2022 season. The test site was selected at a point ∼12 km south of the Chinese Zhongshan Station on the flank of the Dålk Glacier in East Antarctica. Even though the site demonstrates cold-based conditions with temperature at the ice-sheet base of near −5 ∘C, it was thought to be enough for the principal testing of RECAS functionality.
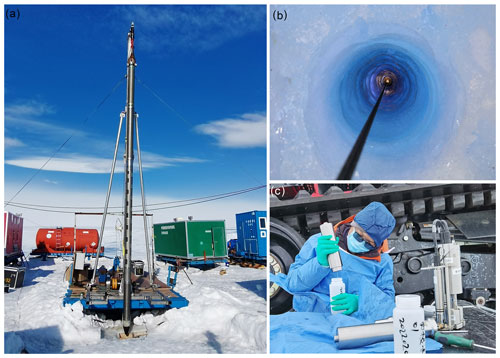
Figure 3RECAS field tests in Antarctica near the Chinese Zhongshan Station, January 2022. (a) The RECAS sonde (in the foreground) is ready for deployment. (b) Sonde on the way to the base of the ice sheet: because of the surface crevassing, meltwater drained from the borehole and down to the depth of approximately 11 m remained dry. (c) Sample of basal ice water is poured from sterile pressurized bottles.
After prior preparations including the camp construction, sonde assembly and debugging, the drilling of the test hole was started on 18 January (Fig. 3). At a depth of 40.5 m, we observed that the temperature sensor of the upper melting tip and load sensor worked abnormally, and the inner winch stopped working. Since the borehole was not yet frozen, the sonde was lifted to the surface for maintenance. A temperature-sensor leakage was found, resulting in a short failure of 12 V power supply circuit and the damage of the inner winch control circuit board. After repairing, the sonde was lowered into the borehole again, and in 103 h, the base of the ice sheet was reached at the final depth of 200.3 m. The base of the ice was recognized at the point when RECAS penetration ultimately stopped. The final depth was about 2 m deeper than the depth of the IBED (ice and bedrock electromechanical drill) borehole drilled to the bedrock by an electromechanical drill ∼60 m to the northeast in 2019 (Talalay et al., 2021).
At the base, water samples were taken into two 360 mL sterilized sample bottles and the following parameters of melted water were recorded: pressure 1.69 MPa, temperature 6.7 ∘C and conductivity 0.0299 mS cm−1. The sampling system was triggered manually. When sampling and monitoring were complete, the sonde began to recover itself to the surface by spooling the cable and melting overlying ice. The total surface input power during drilling was 10.11 kW, including 6.15 kW for melting tips, 2.45 kW for lateral heaters, 1.43 kW cable losses and 0.08 kW for measurement and control system. The average downward penetration rate was 1.85 m h−1, and the average upward penetration rate was 2.94 m h−1. The borehole inclination was in the range of 1.1–1.6∘.
During field tests, RECAS systems worked within the expected range of power consumption. The downward penetration rate slightly exceeded our primary estimations, apparently because the efficiency of melting tips is higher than it was estimated. The upward penetration rate in the freshly frozen ice was predictably higher than the downward penetration rate. The tests revealed a few weak places in the sonde design – hermiticity of the sensors, performance of the cable tension sensor, evenness of the level winder moving, all of which have to be modified in the future. In addition, plans are underway to increase the drilling depth of the sonde and enhance the means of stabilization. Moreover, we are also working to extend the capability of safe and fast drilling in firn and dusty ice.
The water quality sensors performed well and the ability to capture and return water samples provides additional utility to other subglacial sampling systems trialed to date. Although the dust content, salinity, temperature and radiation vary significantly between Earth and extraterrestrial bodies, subglacial lakes in Antarctica are considered to be close analogs of subsurface layers of Mars and icy satellites (such as Titan, Enceladus and Europa). The recent thermal probes for extraterrestrial investigations do not have a well-designed system to recover probes to the surface after deployment (Bar-Cohen and Zacny, 2021). Successful RECAS tests provide a good example of how extraterrestrial probes can be returned to the surface in a safe and reliable manner, although the RECAS cannot be applied in studies of extraterrestrial bodies directly.
Next, we plan to penetrate the active subglacial lake covered by 540 m thick ice at the Flade Isblink ice cap in the northeast of Greenland. Another potential target is subglacial Lake Qilin (∼42 km in length), discovered in the world's biggest canyon system under Antarctic ice in the Princess Elizabeth Land. The lake is overlain with an average ice thickness of 3600 m. Because the lake is very deep, it is proposed to access the lake in two stages. The first stage would include drilling the pilot hole to a depth of 3100–3300 m with the clean hot-water drilling system, providing an access hole with at least 250 mm in diameter. During the second stage, the rest of the 400–600 m thick “ice bridge” will be penetrated by the RECAS. When the sonde reaches the ice-sheet base, the subglacial lake remains isolated from the surface allowing us to take clean water samples. During the RECAS deployment, the main hole is kept open with the required diameter by regular hot-water reaming. However, the tangling of the RECAS cable and the reamer hose would be a concern. As the first step, we plan to test anti-wrap fixture suggested by Blake and Price (2002).
The RECAS and its field operation are relatively cheap: it is estimated to be 10–20 times less expensive than penetration with a hot-water drilling system, while the installation and operation requires only four specialist staff members. The successful tests proved reliability of the new “spider” drilling approach for subglacial lake measurement and sampling, and, accordingly, we believe that the RECAS deployment could begin a new chapter in subglacial lake studies in Antarctica, Greenland and other regions.
The technological and working parameters obtained during RECAS laboratory and field tests are available from the corresponding authors upon reasonable request.
YS led the project and controlled the sonde development. PGT was responsible for the general sonde designing. BL and XF conducted all field and laboratory tests of the sonde. YuL designed the field testing plan. GL, HY, HL, DW, NZ, DG, RW, and YaL designed and tested different parts of the sonde – power supply system, monitoring and control system, melting tips, measuring sensors, etc. PGT and XF wrote the paper with input and ideas from all co-authors.
The contact author has declared that none of the authors has any competing interests.
Publisher's note: Copernicus Publications remains neutral with regard to jurisdictional claims in published maps and institutional affiliations.
We would like to thank specialists involved in the project from the Polar Research Center, Jilin University, Changchun; Polar Research Institute of China, Shanghai; Nanjing Institute of Astronomical Optics and Technology, National Astronomical Observatories, CAS, Nanjing; College of Electronics and Information, Hangzhou Dianzi University; Aerospace System Engineering Shanghai (805), Shanghai; and the National Ocean Technology Center, Tianjin. The project would not be accomplished without their continuous support and valuable help. We thank the Chinese Antarctic Research Expedition (CHINARE) for logistical and financial support of field operations in Antarctica. We are also grateful to the scientific editor, Elizabeth Bagshaw, and reviewers, Kris Zacny, Bernd Dachwald, and Paul Anker, for their insightful comments and suggestions.
This research has been supported by several Chinese government and local agencies with the main funding by the National Key Research and Development Program of the Ministry of Science and Technology of China (project no. 2016YFC1400302) and the National Natural Science Foundation of China (project nos. 41941005 and 41706214).
This paper was edited by Elizabeth Bagshaw and reviewed by Kris Zacny, Bernd Dachwald, and Paul Anker.
Alekhina, I., Ekaykin, A., Moskvin, A., and Lipenkov, V.: Chemical characteristics of the ice cores obtained after the first unsealing of subglacial Lake Vostok, in: Exploration of Subsurface Antarctica: Uncovering Past Changes and Modern Processes, Siegert, M. J., Jamieson, S. S. R., and White, D. A., Geological Society, London, Special Publications, 461, 187, https://doi.org/10.1144/SP461.3, 2017.
Bar-Cohen, Y. and Zacny, K. (Eds): Advances in extraterrestrial drilling ground, ice, and underwater, vol. II, Advances in terrestrial and extraterrestrial drilling: ground, ice, and underwater, CRC Press, Taylor & Francis Group, LLC, 2021.
Blake, E. W. and Price, B.: A proposed sterile sampling system for Antarctic subglacial lakes, Mem. Natl. Inst. Polar Res., 56, 253–263, 2002.
Fleckenstein, W. W. and Eustes, A. W.: Subglacial Antarctic lake environment access methodology: a keystone in a changing world, in: Proceedings of the ISAES X, USGS Open-File Report 2007-1047, Extended Abstract 033, 4 pp., 2007.
Li, Y., Talalay, P. G., Sysoev, M. A., Zagorodnov, V. S., Li, X., and Fan, X.: Thermal heads for melt drilling to subglacial lakes: Design and testing, Astrobiology, 20, 1–15, https://doi.org/10.1089/ast.2019.2103, 2020.
Livingstone, S. J., Li, Y., Rutishauser, A., Sanderson, R. J., Winter, K., Mikucki, J. A., Björnsson, H., Bowling, J. S., Chu, W., Dow, C. F., Fricker, H. A., McMillan, M., Ng, F. S. L., Ross, N., Siegert, M. J., Siegfried, M., and Sole, A. J.: Subglacial lakes and their changing role in a warming climate, Nat. Rev. Earth Environ., 3, 106–124, https://doi.org/10.1038/s43017-021-00246-9, 2022.
Lukin, V. V. and Vasiliev, N. I.: Technological aspects of the final phase of drilling borehole 5G and unsealing Vostok Subglacial Lake, East Antarctica, Ann. Glaciol., 55, 83–89, https://doi.org/10.3189/2014AoG65A002, 2014.
Makinson, K., Anker, P. G. D., Garcés, J., Goodger, D. J., Polfrey, S., Rix, J., Silva, A., Smith, A. M., Uribe, J. A., and Zamor, R.: Development of a clean hot water drill to access Subglacial Lake CECs, West Antarctica, Ann. Glaciol., 62, 250–262, https://doi.org/10.1017/aog.2020.88, 2021.
Pattyn, F.: Antarctic subglacial conditions inferred from a hybrid ice sheet/ice stream model, Earth Planet. Sc. Lett., 295, 451–461, https://doi.org/10.1016/j.epsl.2010.04.025, 2010.
Pearce, D.: Antarctic subglacial lake exploration: a new frontier in microbial ecology, ISME J., 3, 877–880, https://doi.org/10.1038/ismej.2009.53, 2009.
Peng, S., Jiang, X., Tang, Y., Li, C., Li, X., Huang, S., Zhu, T., Shi, J., Sun. Y., Talalay P., Fan, X., Zhang, N., Li, B., Gong, G., and Yu, H.: Recoverable autonomous sonde for subglacial lake exploration: electronic control system design, Ann. Glaciol., 62, 263–279, https://doi.org/10.1017/aog.2021.1, 2021.
Philberth, K.: The thermal probe deep-drilling method by EGIG in 1968 at Station Jarl-Joset, Central Greenland, in: Ice-core drilling: proceeding of the symposium, edited by: Splettstoesser, J. F., 28–30 August 1974, University of Nebraska, Lincoln, USA, University of Nebraska Press, Lincoln, 117–132, 1976.
Priscu, J. C., Kalin, J., Winans, J., Campbell, T., Siegfried, M. R., Skidmore, M., Dore, J. E., Leventer, A., Harwood, D. M., Duling, D., Zook, R., Burnett, J., Gibson, D., Krula, E., Mironov, A., McManis, J., Roberts, G., Rosenheim, B. E., Christner, B. C., Kasic, K., Fricker, H. A., Lyons, W. B., Barker, J., Bowling, M., Collins, B., Davis, C., Gagnon, A., Gardner, C., Gustafson, C., Kim, O.-S., Li, W., Michaud, A., Patterson, M. O., Tranter, M., Venturelli, R., Vick-Majors, T., Elsworth, C., and The SALSA Science Team: Scientific access into Mercer Subglacial Lake: scientific objectives, drilling operations and initial observations, Ann. Glaciol., 62, 340–352, https://doi.org/10.1017/aog.2021.10, 2021.
Shi, J., Huang, S., Wang, B., Li, C., Peng, S., Sun, Y., Talalay, P., and Yu, H.: Design and analysis of deepwater tension sensors for ice drill application, Ann. Glaciol., 62, 46–52, https://doi.org/10.1017/aog.2020.71, 2021.
Siegert, M. J., Clarke, R. J., Mowlem, M., Ross, N., Hill, C. S., Tait, A., Hodgson, D., Parnell, J., Tranter, M., Pearce, D., Bentley, M. J., Cockell, C., Tsaloglou, M.-N., Andy Smith, A., Woodward, J., Brito, M. P., and Waugh, E.: Clean access, measurement, and sampling of Ellsworth Subglacial Lake: a method for exploring deep Antarctic subglacial lake environments, Rev. Geophys., 50, RG1003, https://doi.org/10.1029/2011RG000361, 2012.
Siegert, M. J., Makinson, K., Blake, D., Mowlem, M., and Ross, N.: An assessment of deep hot-water drilling as a means to undertake direct measurement and sampling of Antarctic subglacial lakes: experience and lessons learned from the Lake Ellsworth field season 2012/13, Ann. Glaciol., 55, 59–73, https://doi.org/10.3189/2014AoG65A008, 2014.
Siegert, M. J., Ross, N., and Le Brocq, A. M.: Recent advances in understanding Antarctic subglacial lakes and hydrology, Phil. Trans. R. Soc. A, 374, 20140306, https://doi.org/10.1098/rsta.2014.0306, 2016.
Talalay, P., Li, X., Zhang, N., Fan, X., Sun, Y., Cao, P., Wang, R., Yang, Y., Liu, Y., Liu, Y., Wu, W., Yang, C., Hong, J., Gong, D., Zhang, H., Li, X., Chen, Y., Liu, A., and Li, Y.: Antarctic subglacial drill rig. Part II: Ice and Bedrock Electromechanical Drill (IBED), Ann. Glaciol., 62, 12–22, https://doi.org/10.1017/aog.2020.38, 2021.
Talalay, P. G.: Thermal ice drilling technology, Geological Publishing House and Springer Nature Singapore Pte Ltd., Singapore, 2020.
Talalay, P. G., Zagorodnov, V. S., Markov, A. N., Sysoev, M. A., and Hong, J.: Recoverable autonomous sonde (RECAS) for environmental exploration of Antarctic subglacial lakes: general concept, Ann. Glaciol., 55, 23–30, https://doi.org/10.3189/2014AoG65A003, 2014.
Tulaczyk, S., Mikucki, J. A., Siegfried, M. R. Priscu, J. C., Barcheck, C. G., Beem, L. H., Behar, A., Burnett, J., Christner, B. C., Fisher, A. F., Fricker, H. A., Mankoff, K. D., Powell, R. D., Rack, F., Sampson, D., Scherer, R. P., Schwartz, S. Y., and The Wissard Science Team: WISSARD at Subglacial Lake Whillans, West Antarctica: Scientific operations and initial observation, Ann. Glaciol., 55, 51–58, https://doi.org/10.3189/2014AoG65A009, 2014.
Yu, H., Zhu, T., Jiang, X., Tang, Y., Li, X., Li, C., Huang, S., Shi, J., Sun, Y., Talalay, P., Fan, X., Li, X., Li, Y., and Peng, S.: Recoverable Autonomous Sonde for subglacial lakes exploration: heating control system design, Ann. Glaciol., 62, 280–292, https://doi.org/10.1017/aog.2021.5, 2021.
Zhang, N., Liu, H., Talalay, P., Sun, Y., Li, N. Fan, X., Li, B., Gong, D., Hong, J., Wang, T., Liu, A., Li, Y., Liu, Y., Wang, R., Yang, Y., and Wang, L.: New synthetic fiber armored cable for freezing-in thermal ice probes, Ann. Glaciol., 62, 179–190, https://doi.org/10.1017/aog.2020.74, 2021.